Semiconductor 101: A Comprehensive Look at Semiconductor Definition and Use Cases
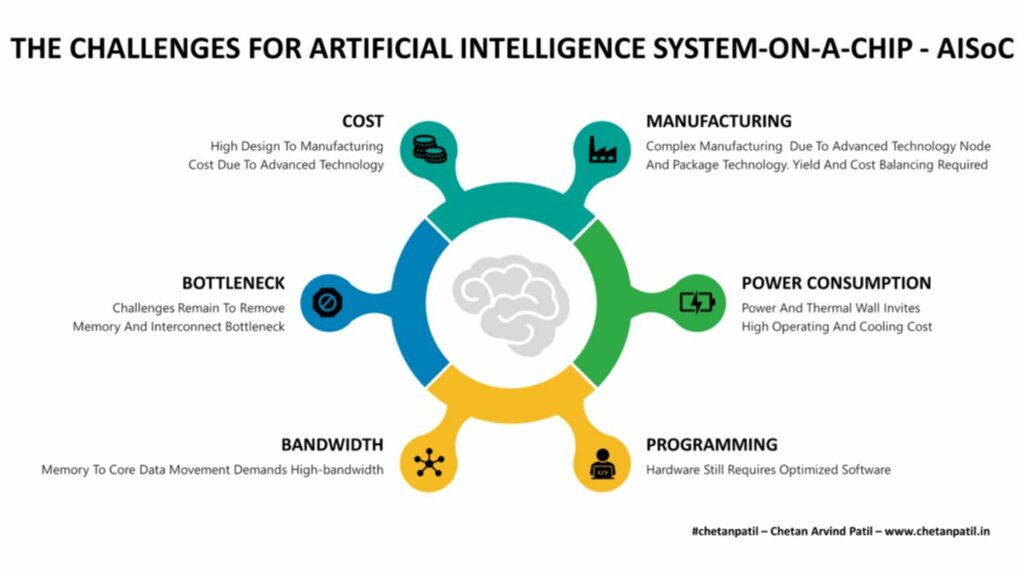
Semiconductors are fundamental components that drive the modern digital era, integral to everything from consumer electronics to advanced automotive systems. Understanding their composition, characteristics, and applications is crucial for grasping how these tiny yet powerful devices shape our world. As the semiconductor industry experiences a renaissance, propelled by emerging technologies like generative AI and electric vehicles, it’s important to delve into the intricacies of semiconductor definition and use cases.
Key Takeaways
- Semiconductors, made primarily of metalloids like silicon and germanium, are the building blocks of modern electronics, with evolving materials like graphene offering future advancements.
- Key semiconductor devices include transistors (BJTs, FETs, MOSFETs, IGBTs), each with unique properties and applications in various electronic circuits.
- The semiconductor industry is a pivotal economic force with complex supply chains, significant investment opportunities, and is influenced by the rise of generative AI and electric vehicles.
- Semiconductor manufacturing and engineering face challenges in mass production and innovation, with companies striving to overcome limitations while scaling up operations.
- Future semiconductor trends involve the continued miniaturization of devices, the potential of optical transistors, and the enduring influence of Moore’s Law on technological progress.
Understanding Semiconductors: Composition and Characteristics
The Role of Metalloids in Semiconductor Technology
Metalloids, particularly germanium (Ge) and silicon (Si), have played a pivotal role in the development of semiconductor technology. Germanium was the first to be used in 1947, marking the beginning of the semiconductor era, followed by silicon in 1954. These elements are valued for their ability to conduct electricity under certain conditions, making them essential for various electronic devices.
The unique properties of metalloids allow them to act as conductors or insulators, depending on the presence of impurities, known as dopants. This characteristic is harnessed in devices like Metal–Semiconductor FETs (MESFETs) and High-Electron-Mobility Transistors (HEMTs), which are especially suitable for high-frequency applications.
Here is a comparison of electron mobility in different semiconductor materials, which is a measure of how quickly charge carriers (electrons) can move through the material when an electric field is applied:
Material | Electron Mobility (cm^2/Vs) |
---|---|
Germanium (Ge) | 3900 |
Silicon (Si) | 1500 |
Gallium Arsenide (GaAs) | 8500 |
Despite germanium’s higher electron mobility, silicon has become the predominant material due to its superior thermal stability, abundance, and the maturity of silicon-based manufacturing processes.
Compound Semiconductors and Their Applications
Compound semiconductors are integral to the advancement of various technologies. Unlike pure elemental semiconductors like silicon, compound semiconductors are composed of two or more elements, such as gallium arsenide (GaAs) and silicon carbide (SiC). These materials exhibit distinct electrical properties that make them suitable for specific applications where silicon might not perform as well.
Compound semiconductors offer significant benefits for green hydrogen applications, particularly in the field of renewable energy and electric vehicles. For instance, gallium arsenide is renowned for its high electron mobility, which is crucial for high-frequency and high-efficiency devices. Silicon carbide, on the other hand, is valued for its high thermal conductivity and ability to withstand high temperatures, making it ideal for power electronics.
The table below summarizes some key characteristics of common compound semiconductors:
Material | Junction Forward Voltage @ 25°C (V) | Electron Mobility @ 25°C (m2/(V·s)) | Hole Mobility @ 25°C (m2/(V·s)) | Max. Junction Temp. (°C) |
---|---|---|---|---|
Ge | 0.27 | 0.39 | 0.19 | 70 to 100 |
Si | 0.71 | 0.14 | 0.05 | 150 to 200 |
GaAs | 1.03 | 0.85 | 0.05 | 150 to 200 |
Al–Si | 0.3 | — | — | 150 to 200 |
As the semiconductor industry continues to evolve, the role of compound semiconductors becomes increasingly critical, powering new movements like generative AI and electric vehicles.
Emerging Materials: Graphene and Beyond
The exploration of emerging materials in semiconductor technology is advancing rapidly, with graphene at the forefront. This atomically thin layer of carbon atoms arranged in a hexagonal lattice has demonstrated exceptional electrical, thermal, and mechanical properties. Researchers have unveiled the world’s first functional semiconductor made from graphene, which could revolutionize the industry with its potential for ultrafast electronics and high sensitivity.
Graphene’s atomic structure provides a protective mechanism that allows for the processing of ultrathin electronic components in various chemical environments. This discovery has opened the door to the use of other 2D materials, such as MXenes, which are transition metal nitrides, carbonitrides, or carbides with promising applications in areas like micromirrors due to their reflective properties.
The table below summarizes some of the key properties and potential applications of these emerging materials:
Material | Key Properties | Potential Applications |
---|---|---|
Graphene | High electrical conductivity, thermal stability | Ultrafast electronics, photodetectors |
MXenes | Reflective, mechanically robust | Micromirrors, sensors |
As the search for new van der Waals materials continues, the semiconductor industry eagerly anticipates the integration of these novel materials into next-generation devices.
Semiconductor Devices and Their Mechanisms
The Evolution of Transistors: From BJTs to FETs
The transistor has undergone significant evolution since its inception. Initially, the bipolar junction transistor (BJT) was the cornerstone of semiconductor devices, capable of amplifying or switching electrical signals. However, BJTs faced challenges in mass production due to their complexity and size.
The field-effect transistor (FET) emerged as a promising alternative, offering a different mechanism of operation. Unlike BJTs, which control current using a small current at the base, FETs use a voltage at the gate to control the current between the source and drain. This distinction has led to a variety of FETs, including the JFET and MOSFET, each with unique characteristics and applications.
The MOSFET, in particular, has become the most widely used type of transistor, heralding a new era in electronics with its ability to enable smaller, cheaper, and more efficient devices. Its invention marked a significant achievement in the field, allowing for the miniaturization of electronic components and the proliferation of modern electronic devices.
MOSFETs and Their Significance in Modern Electronics
The metal-oxide-semiconductor field-effect transistor (MOSFET) has revolutionized the world of electronics. Its ability to amplify or switch electronic signals by changing conductivity with applied voltage has made it indispensable in modern technology. The insulated gate of a MOSFET, which controls the device’s conductivity, is a key feature that allows for precise control over electronic functions.
MOSFETs are celebrated for their scalability, which has been crucial in the miniaturization of electronic devices. This characteristic has enabled the production of highly integrated circuits, with tens of thousands of transistors on a single chip. The table below illustrates the impact of MOSFETs on the density of integrated circuits over time:
Year | Transistors per IC |
---|---|
1970 | 1,000 |
1980 | 10,000 |
1990 | 100,000 |
2000 | 1,000,000 |
2010 | 10,000,000 |
In addition to their role in digital circuits, MOSFETs are also used in various analog applications, such as voltage regulators and amplifiers. Their dominance in the market is attributed to their low power consumption and high density compared to bipolar junction transistors. The automation of MOSFET production has led to incredibly low costs per transistor, further cementing their place in the industry.
Insulated-Gate Bipolar Transistors (IGBTs) and Their Uses
Insulated-Gate Bipolar Transistors (IGBTs) represent a fusion of two technologies: the high input impedance of Metal-Oxide-Semiconductor Field-Effect Transistors (MOSFETs) and the high switching speeds of Bipolar Junction Transistors (BJTs). This combination allows IGBTs to control large amounts of power with minimal input energy, making them ideal for heavy-duty industrial applications.
IGBTs are commonly used in applications requiring high voltage and current, such as electric locomotives, induction heating, and variable-frequency drives. A notable example is the ASEA Brown Boveri (ABB) 5SNA2400E170100, which contains three n–p–n IGBTs, each capable of handling significant electrical loads.
IGBT Model | Voltage Rating (V) | Current Rating (A) | Dimensions (mm) | Weight (kg) |
---|---|---|---|---|
5SNA2400E170100 | 1,700 | 2,400 | 38 x 140 x 190 | 1.5 |
The integration of power diodes in IGBTs enhances their efficiency and reliability, particularly in circuits where reverse current flow may occur. As the semiconductor industry continues to evolve, the role of IGBTs is expected to expand, especially in sectors focused on energy efficiency and high-power solutions.
The Semiconductor Industry: An Economic Overview
Supply Chain Dynamics and Key Players
The semiconductor supply chain is a complex and multifaceted ecosystem, involving a wide array of companies that specialize in different stages of chip production. From design and engineering to manufacturing and distribution, each player has a critical role in bringing semiconductor devices to market. Key players in the industry often have competitive advantages that enable them to dominate certain segments of the market.
The following table highlights some of the top semiconductor companies and their respective market positions:
Rank | Company Name | Notable Segment |
---|---|---|
#1 | Taiwan Semiconductor Manufacturing Co. | Foundry Services |
#2 | NVIDIA Corp. | Graphics and AI Processors |
#3 | Intel Corp. | Microprocessors and Chipsets |
#4 | Broadcom Inc. | Broadband and Wireless Chips |
Understanding the dynamics of the supply chain is crucial for investors looking to navigate the semiconductor market. The industry is known for its cyclical nature, with demand for chips often influenced by broader economic trends and technological advancements. As such, companies must be agile and responsive to shifts in market conditions to maintain their competitive edge.
Investment Opportunities and Competitive Advantages
The semiconductor industry presents a dynamic landscape for investors, with several companies showcasing strong potential for growth. Investment considerations must account for innovation, market position, and financial health.
Key factors influencing investment decisions include product portfolios, cash reserves, and strategic acquisitions. For instance, a company’s ability to innovate and diversify its product offerings can significantly enhance its competitive edge and attract investor interest.
- Product Portfolio: A diverse range of innovative semiconductor products can drive a company’s growth.
- Cash Position: Strong cash reserves enable strategic investments and weathering of market downturns.
- Acquisition Potential: The capacity for acquiring complementary businesses can lead to market expansion and consolidation.
Despite potential challenges such as share dilution and complex shareholder structures, companies that navigate these complexities successfully often emerge stronger. Investors are advised to stay informed about industry cycles and company-specific developments to make well-rounded investment decisions.
Impact of Generative AI and Electric Vehicles on the Industry
The semiconductor industry is experiencing a transformative phase with the advent of generative AI and the surge in electric vehicle (EV) production. Generative AI’s demand for advanced chips is pushing semiconductor companies to innovate, while the EV market’s growth is reshaping supply chains.
- Generative AI requires high-performance semiconductors to process complex algorithms, leading to increased investment in R&D.
- The EV sector’s reliance on semiconductors for battery management and power control systems is intensifying the focus on reliability and efficiency.
Tesla’s foray into lithium refining, aimed at reducing EV battery costs, exemplifies the industry’s vertical integration trend. Meanwhile, companies like Nvidia are capitalizing on AI’s growth, with their chips powering a new generation of data centers. The ripple effects of these developments are felt throughout the industry, influencing stock valuations and investment strategies.
Semiconductor Manufacturing and Engineering
The Process of Creating Semiconductor Devices
The fabrication of semiconductor devices is a complex and nuanced process, pivotal to the advancement of modern technology. Semiconductor device fabrication is the intricate procedure employed to create the integrated circuits (ICs) that are the building blocks of electronic devices such as computer processors and smartphones.
The journey begins with the creation of a silicon wafer, which undergoes a series of steps known as surface passivation. This critical technique involves coating the wafer with an insulating layer of silicon oxide, allowing electricity to reliably reach the semiconducting silicon layer beneath. The process was a significant breakthrough, enabling the mass-production of silicon integrated circuits and paving the way for the metal-oxide-semiconductor (MOS) process.
Following the initial preparation, the semiconductor material is carefully inserted into a glass tube and heated to high temperatures. This allows the material to be drawn into a thin, continuous strand, a process akin to pulling molten glass into fibers. The differing melting points and thermal expansion rates of the materials involved play a crucial role in this step, ensuring the semiconductor fills the space within the glass container as it melts and solidifies into the desired shape.
Challenges in Mass-Producing Semiconductors
The mass-production of semiconductors is a complex and intricate process, fraught with challenges that can impact both the quality and quantity of output. Ensuring the integrity of semiconductor fibers is paramount, as they must remain flexible and defect-free for stable signal transmission. Unfortunately, current manufacturing methods often induce stress and instability, leading to cracks and deformities in the semiconductor cores. This not only affects performance but also hampers further development.
Another significant hurdle is the environmental impact of semiconductor fabrication. The process involves various chemicals, and the construction and operation of fabrication plants, or ‘fabs’, can lead to environmental consequences. Companies must navigate these issues while striving to maintain efficiency and minimize waste.
The relentless drive for miniaturization presents its own set of challenges. As transistors, the building blocks of semiconductor devices, shrink to atomic scales, the limitations of silicon technology become apparent. The industry is thus compelled to explore alternative materials with new properties to push the boundaries of technology further.
Innovations in Semiconductor Engineering
The relentless pursuit of innovation in semiconductor engineering has led to remarkable advancements in the materials and processes used to create these critical components. In an era where technology evolves at an unprecedented pace, the semiconductor industry stands as the backbone of innovation, driving progress across various sectors.
One of the pivotal innovations was the introduction of surface passivation by Mohamed Atalla. This process involved coating a silicon wafer with an insulating layer of silicon oxide, which allowed electricity to reliably penetrate the semiconducting silicon below. This breakthrough paved the way for the mass-production of silicon integrated circuits, revolutionizing the industry.
The evolution of semiconductor materials has also been significant. From the initial use of germanium and silicon to the incorporation of compounds like gallium arsenide and silicon carbide, each material has contributed to the scaling of transistors and the enhancement of device performance. The ongoing research into materials such as graphene promises to further push the boundaries of what is possible in semiconductor engineering.
Here is a brief overview of key semiconductor materials and their first year of use:
Material | First Use Year |
---|---|
Germanium | 1947 |
Silicon | 1954 |
Gallium Arsenide | 1966 |
Silicon Carbide | 1997 |
Silicon-Germanium Alloy | 1989 |
Graphene (Research Ongoing) | 2004 |
These materials, along with innovative fabrication techniques, continue to shape the future of the semiconductor industry.
Future Trends and Developments in Semiconductors
The Influence of Moore’s Law on Semiconductor Evolution
The relentless progression of semiconductor technology has been largely guided by Moore’s Law, an empirical observation made by Gordon Moore in 1965. Moore’s Law refers to the prediction that the number of transistors on a single chip would double approximately every two years, enhancing performance while keeping costs down. This principle has driven the industry to innovate continuously, shrinking transistors to microscopic sizes.
As we approach the physical limitations of silicon-based technology, the industry faces significant challenges. Transistors are now reaching sizes where quantum effects and other physical phenomena can interfere with their operation. The search for new materials and techniques is vital to maintain the pace of advancement.
Despite these challenges, the semiconductor industry has consistently found ways to adapt and extend the relevance of Moore’s Law. The table below illustrates the historical trend of transistor count doubling, showcasing the law’s impact over the decades:
Year | Transistor Count (approximate) |
---|---|
1971 | 2,300 |
1975 | 6,000 |
1980 | 20,000 |
1985 | 75,000 |
1990 | 275,000 |
1995 | 1,000,000 |
2000 | 42,000,000 |
2005 | 291,000,000 |
2010 | 2,300,000,000 |
2015 | 7,200,000,000 |
The future of semiconductors may hinge on breakthroughs in materials science and engineering, as the industry seeks to overcome the barriers imposed by the laws of physics. The evolution of semiconductors will continue to be a testament to human ingenuity and the relentless pursuit of progress.
Nanotechnology and the Miniaturization of Semiconductors
The relentless pursuit of miniaturization in semiconductor technology has led to the creation of transistors that are mere atoms in width. This trend, mirroring the broader movement towards miniaturization in various technological fields, is pushing the boundaries of current silicon-based methods. As we approach the physical limits of silicon, the industry is compelled to explore alternative materials that can sustain the trajectory of rapid technological progress.
Nanotechnology has played a pivotal role in this downsizing journey. For instance, the development of nanoscale vacuum-channel transistors represents a significant leap forward. These transistors, which are only 150 nanometers in size, can be produced using standard silicon processing techniques, yet they offer high-speed operation and the potential for low power consumption. Such innovations are not only impressive in their technical specifications but also in their ability to withstand extreme environments.
The miniaturization process also brings forth new challenges and opportunities in the manufacturing of semiconductor devices. Advanced materials and fabrication techniques, such as nanoimprints and 3D imprints, are under intense development. These methods are expected to drive innovation and enable the creation of novel devices, including micro-opto-electro-mechanical systems (MOEMS) that operate at the microscale and nanoscale levels.
Potential Breakthroughs: Optical Transistors and Beyond
The quest for the next leap in semiconductor technology is leading to the exploration of optical transistors. These devices, which use light rather than electrons to transmit signals, promise to revolutionize data processing speeds and energy efficiency. The development of optical transistors aligns with the broader industry trend towards silicon photonics, a field that has seen significant growth due to its applications in optical communications.
A variety of novel materials and concepts are being investigated to overcome the limitations of current semiconductor devices. Among these, carbon-based materials like graphene, as well as advanced compounds such as aluminum nitride and diamond, are showing potential for high-performance transistors. The table below outlines some of the emerging transistor types and their distinctive properties:
Transistor Type | Material | Notable Feature |
---|---|---|
Optical Transistor | Various | Light-based signal transmission |
Carbon-doped Si-Ge:C | Silicon-Germanium | Enhanced electrical properties |
Diamond Transistor | Diamond | High thermal conductivity |
As the industry continues to innovate, the integration of these advanced materials into semiconductor manufacturing could lead to devices that are not only faster and more reliable but also capable of operating in extreme conditions. This would have profound implications for a range of applications, from space exploration to quantum computing.
Conclusion
In conclusion, semiconductors are not just components; they are the lifeblood of our digital era, fueling advancements from AI to electric vehicles. Our journey through ‘Semiconductor 101’ has illuminated the intricate structures and materials that define these technological marvels, from the pioneering use of germanium to the modern dominance of silicon and the promising potential of gallium arsenide and silicon-germanium alloys. We’ve explored the complex supply chain and the competitive landscape that shapes the semiconductor industry, highlighting the importance of understanding the nuances of this market for investors and enthusiasts alike. As we stand on the cusp of new technological revolutions, the role of semiconductors becomes ever more critical. It’s an exciting time to delve into the semiconductor world, and we hope this comprehensive look has provided a solid foundation for your continued exploration and appreciation of this pivotal field.
Frequently Asked Questions
What are semiconductors and why are they important?
Semiconductors are materials with electrical conductivity between that of a conductor and an insulator. They are crucial for creating electronic components like transistors and diodes, and are the backbone of modern electronic devices, from smartphones to electric vehicles.
What is the role of metalloids in semiconductor technology?
Metalloids, such as germanium and silicon, have properties between metals and non-metals, making them ideal for controlling electrical conductivity in semiconductors. They are commonly used as a base material in semiconductor devices.
How have transistors evolved over time?
Transistors have evolved from the bulky junction transistor to more efficient and compact forms like Field-Effect Transistors (FETs), including MOSFETs, which are now widely used in modern electronics due to their low power consumption and high switching speeds.
What impact do generative AI and electric vehicles have on the semiconductor industry?
Generative AI and electric vehicles are driving demand for advanced semiconductors, leading to growth in the industry. They require powerful and efficient chips, which spurs innovation and investment in semiconductor research and manufacturing.
What are the main challenges in semiconductor manufacturing?
Semiconductor manufacturing faces challenges such as the complexity of the production process, the need for high precision and cleanliness, and the significant investment in research and development to keep up with technological advancements.
What are some future trends in semiconductor technology?
Future trends include further miniaturization of semiconductor devices through nanotechnology, the development of optical transistors for faster data transmission, and the continuous push for more efficient and powerful chips in line with Moore’s Law.