Semiconductor Design: Key Techniques and Challenges
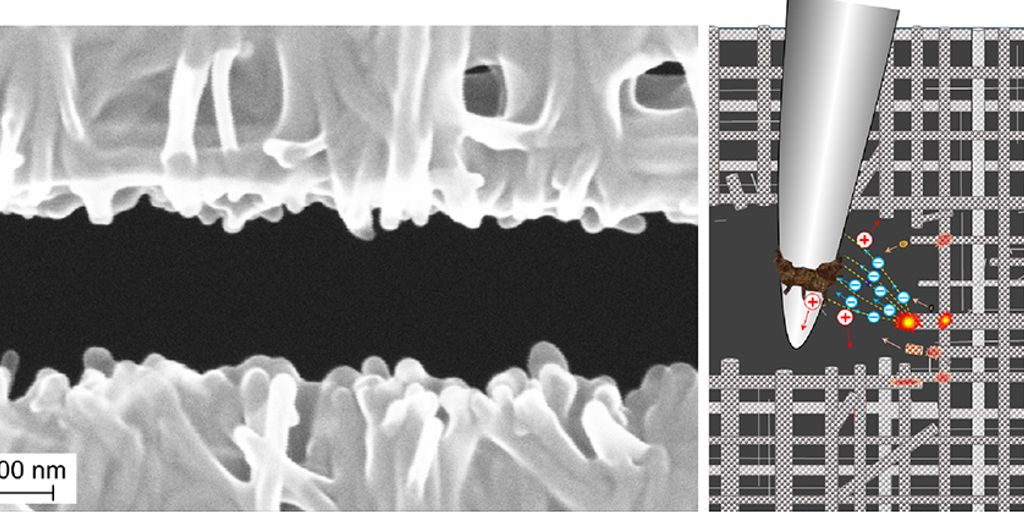
Semiconductor design services, the cornerstone of modern electronics, face a myriad of challenges driven by the relentless pursuit of smaller, faster, and more power-efficient devices. These challenges are rooted in rigorous research and industry experience, shaping the landscape of semiconductor design. In this article, we explore the key research-driven challenges that semiconductor design service providers encounter in today’s highly competitive environment.
Key Takeaways
- Advanced techniques such as High-Level Synthesis, Design for Testability, and Low-Power Design are crucial for modern semiconductor design.
- Shrinking process nodes present significant challenges, including Moore’s Law limitations, increased development costs, and manufacturing complexity.
- Power efficiency and thermal management are critical, with techniques like Dynamic Voltage Scaling, Thermal-Aware Design, and Energy Harvesting playing vital roles.
- AI is revolutionizing semiconductor design through AI-driven design automation, predictive maintenance, and enhanced simulation accuracy.
- Security concerns such as Hardware Trojans, Side-Channel Attacks, and the need for secure design practices are increasingly important in semiconductor design.
Advanced Techniques in Semiconductor Design
High-Level Synthesis
High-Level Synthesis (HLS) is a transformative approach in semiconductor design. It allows designers to work at a higher level of abstraction, using languages like C++ or SystemC, which are then automatically translated into hardware description languages. This technique significantly reduces the design cycle time and enhances productivity.
Design for Testability
Design for Testability (DFT) is crucial for ensuring that semiconductor devices can be efficiently tested for defects. By incorporating testability features during the design phase, manufacturers can detect and address issues early, reducing the risk of costly post-production failures. DFT techniques include scan chains, built-in self-test (BIST), and boundary scan.
Low-Power Design
Low-Power Design is essential in today’s energy-conscious world. Techniques such as clock gating, power gating, and multi-threshold CMOS are employed to minimize power consumption without compromising performance. These methods are particularly important for battery-operated devices and applications where power efficiency is critical.
Challenges in Shrinking Process Nodes
Moore’s Law Limitations
As semiconductor technology advances, designers face the challenge of designing circuits on increasingly smaller process nodes. Adhering to Moore’s Law becomes increasingly challenging as the returns in scaling down transistors diminish. This results in skyrocketing development costs and complexity.
Increased Development Costs
The cost of developing new technology nodes is rising exponentially. Research by the Semiconductor Industry Association (SIA) highlights the diminishing returns in scaling down transistors, leading to increased development costs. This is compounded by the need for more sophisticated equipment and materials.
Complexity in Manufacturing
The complexity of manufacturing at smaller process nodes is another significant challenge. Manufacturers face issues such as minimal component reuse and exploding design data sizes, which slow down performance. Additionally, there is a lack of traceability from requirements to design to verification, often triggering re-spins.
Power Efficiency and Thermal Management
In the era of mobile and IoT devices, power efficiency is paramount. Research from the International Technology Roadmap for Semiconductors (ITRS) underscores the struggle to balance performance with power consumption while managing the resulting heat. Innovative thermal management solutions are essential.
Dynamic Voltage Scaling
Dynamic Voltage Scaling (DVS) is a technique used to adjust the voltage supplied to a processor based on the required performance. By lowering the voltage during less demanding tasks, power consumption can be significantly reduced. This technique is crucial for extending battery life in mobile devices.
Thermal-Aware Design
Thermal-aware design involves creating circuits and systems that can efficiently manage heat dissipation. This includes the use of materials with better thermal conductivity and the strategic placement of components to minimize hotspots. Effective thermal management ensures that devices operate within safe temperature ranges, thereby enhancing reliability and performance.
Energy Harvesting Techniques
Energy harvesting techniques involve capturing and storing energy from external sources such as solar, thermal, or kinetic energy. These techniques are particularly useful for IoT devices that require long-term, low-power operation. By utilizing ambient energy, these devices can reduce their dependency on traditional power sources, leading to more sustainable and efficient designs.
The Role of AI in Semiconductor Design
AI-Driven Design Automation
AI-driven design automation is revolutionizing the semiconductor industry by automating routine design tasks. This allows engineers to focus on more complex decisions, significantly enhancing productivity. AI-based EDA tools enable more effective design space exploration, which is crucial for meeting stringent design targets. However, the reliance on machine learning algorithms results in a substantial increase in design data, often overwhelming traditional data management systems.
Predictive Maintenance
Predictive maintenance leverages AI to foresee potential failures in semiconductor manufacturing equipment. By analyzing historical data and identifying patterns, AI can predict when a machine is likely to fail, allowing for timely maintenance and reducing downtime. This not only improves the efficiency of the manufacturing process but also extends the lifespan of the equipment.
Enhanced Simulation Accuracy
AI enhances simulation accuracy by providing more precise models and predictions. This is particularly important in the verification and testing phases of semiconductor design, where accurate simulations can save time and resources. AI-driven simulations can identify potential issues early in the design process, reducing the need for costly revisions later on.
Security Concerns in Semiconductor Design
Hardware Trojans
The semiconductor manufacturing world faces constant threats from hardware trojans and other security vulnerabilities. These malicious alterations can compromise the integrity and functionality of a chip, making it crucial to implement robust detection and prevention mechanisms.
Side-Channel Attacks
Side-channel attacks exploit indirect information leakage, such as power consumption or electromagnetic emissions, to extract sensitive data from semiconductor devices. Protecting digital lives requires a multi-faceted approach that includes both hardware and software countermeasures.
Secure Design Practices
To safeguard chip security, semiconductor designs must incorporate secure design practices. This includes the use of hardware-based security modules to protect against counterfeiting and reverse engineering. Additionally, stringent access controls and data management systems are essential to prevent unauthorized access to sensitive design data.
Collaboration Across the Semiconductor Ecosystem
Industry-Academia Partnerships
Industry-academia partnerships are crucial for driving innovation in semiconductor design. These collaborations enable the sharing of cutting-edge research and practical industry insights, fostering an environment where theoretical knowledge meets real-world application. Such partnerships often lead to groundbreaking advancements that might not be possible in isolation.
Open-Source Initiatives
Open-source initiatives play a significant role in democratizing semiconductor design. By providing access to design tools and resources, these initiatives lower the entry barriers for startups and smaller companies. This collaborative approach accelerates innovation and allows for a more diverse range of ideas and solutions to emerge.
Standardization Efforts
Standardization efforts are essential for ensuring compatibility and interoperability across different semiconductor components and systems. These efforts involve collaboration among various stakeholders, including industry leaders, regulatory bodies, and academic institutions. Standardization not only simplifies the design process but also enhances the reliability and performance of semiconductor products.
Future Trends in Semiconductor Design
The semiconductor industry is on the brink of several transformative trends that promise to redefine the landscape of technology. Exploring semiconductor technology trends 2024 reveals a future rich with innovation and groundbreaking discoveries.
Quantum Computing
Quantum computing stands at the forefront of future semiconductor design. This technology leverages quantum mechanics to perform computations at unprecedented speeds, potentially solving problems that are currently intractable for classical computers.
Neuromorphic Engineering
Neuromorphic engineering aims to mimic the neural structure of the human brain to create more efficient and powerful computing systems. This approach could revolutionize artificial intelligence and machine learning by providing hardware that is inherently suited for these tasks.
3D Integrated Circuits
3D integrated circuits (3D ICs) involve stacking silicon wafers or dies and interconnecting them vertically. This technique can significantly enhance performance and reduce power consumption, addressing some of the key challenges in modern semiconductor design.
Conclusion
In conclusion, semiconductor design services are at the forefront of technological innovation, but they face formidable challenges rooted in extensive research and industry trends. Navigating these challenges requires a strategic blend of cutting-edge research, innovative methodologies, and collaboration across the semiconductor ecosystem. Successfully addressing these hurdles will be pivotal in shaping the future of semiconductor design and advancing the capabilities of electronic devices across industries. As technology continues to evolve, the role of semiconductor design will only become more critical in meeting the demands of the modern digital age.
Frequently Asked Questions
What are the main challenges in semiconductor design?
The main challenges in semiconductor design include shrinking process nodes, power efficiency, thermal management, increased development costs, and complexity in manufacturing.
How does Moore’s Law impact semiconductor design?
Moore’s Law, which predicts the doubling of transistors on a chip approximately every two years, is becoming harder to maintain due to physical and economic limitations. This results in increased development costs and complexity.
What techniques are used to improve power efficiency in semiconductor design?
Techniques such as dynamic voltage scaling, thermal-aware design, and energy harvesting are commonly used to improve power efficiency in semiconductor design.
How is AI influencing semiconductor design?
AI is revolutionizing semiconductor design through AI-driven design automation, predictive maintenance, and enhanced simulation accuracy, making the design process more efficient and reliable.
What are the security concerns in semiconductor design?
Security concerns in semiconductor design include hardware Trojans, side-channel attacks, and the need for secure design practices to protect against these vulnerabilities.
Why is collaboration important in the semiconductor ecosystem?
Collaboration across the semiconductor ecosystem, including industry-academia partnerships, open-source initiatives, and standardization efforts, is crucial for innovation, cost reduction, and addressing complex design challenges.