Understanding the Different Types of Semiconductors: Materials and Uses
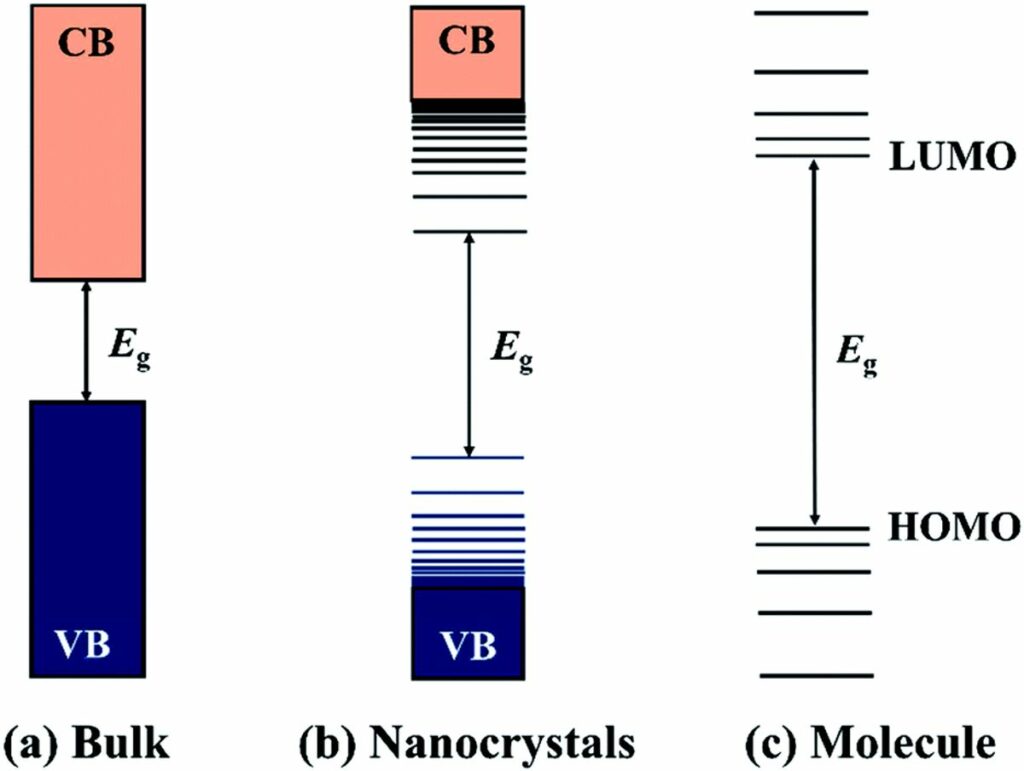
Semiconductors are the cornerstone of modern electronics, bridging the gap between conductors and insulators with their unique ability to control electrical conductivity. This article provides a comprehensive understanding of the different types of semiconductor materials, their characteristics, and their extensive applications across various industries. From the basics of conductivity and band theory to the advanced manufacturing techniques, we explore the pivotal role semiconductors play in shaping the technological landscape.
Key Takeaways
- Semiconductors are materials with controlled conductivity, essential for electronic devices like microprocessors and high-voltage appliances.
- The two main types of semiconductor devices are two-terminal, such as diodes, and three-terminal, such as transistors.
- P-type semiconductors are characterized by an excess of holes and are crucial for devices like LEDs, BJTs, and solar cells.
- Advancements in semiconductor technology include sophisticated manufacturing techniques like lithography and the use of nanotechnology.
- Semiconductor materials and devices are integral to industries including computing, telecommunications, automotive, and renewable energy.
Fundamentals of Semiconductor Materials
Conductivity and Band Theory
At the heart of semiconductor functionality is the concept of conductivity and band theory. Semiconductors possess a unique place on the conductivity spectrum; they are neither perfect conductors like copper nor complete insulators like glass. Instead, they exhibit conductivity that increases with temperature, a property that is crucial for their operation in electronic devices.
The behavior of semiconductors is governed by the band theory of solids. In semiconductors, the valence band and conduction band are separated by an energy gap known as the band gap. At absolute zero (0K), a semiconductor behaves like an insulator because there are no electrons in the conduction band. However, as the temperature rises, electrons gain enough energy to jump across the band gap from the valence band to the conduction band, leading to an increase in conductivity.
Doping is a process that further modifies the conductivity of semiconductors. By introducing impurities into the semiconductor material, the number of charge carriers, either electrons or holes, is increased, enhancing the material’s conductive properties. The following list highlights the effects of temperature and doping on semiconductor conductivity:
- An increase in temperature leads to a higher electron mobility and more charge carriers in the conduction band.
- Doping introduces additional charge carriers, reducing the energy required for electrons to bridge the band gap.
- The resulting increase in conductivity makes semiconductors highly versatile for various electronic applications.
Doping and Charge Carriers
Doping is a critical process in semiconductor technology, involving the intentional introduction of impurities into the semiconductor material to alter its electrical properties. In P-type semiconductors, doping is achieved by adding elements from Group III of the periodic table, such as boron or gallium. These elements have fewer valence electrons than the semiconductor material, leading to the creation of holes which act as positive charge carriers.
The presence of these holes is what distinguishes P-type semiconductors from their N-type counterparts. While N-type semiconductors have an excess of electrons, P-type materials have an excess of holes, resulting in a surplus of positive charges. This difference is crucial for the formation of P-N junctions, which are the foundation of many semiconductor devices.
- Hole Mobility: Holes in P-type semiconductors facilitate the movement of electrical charge, contributing to the material’s conductivity.
- Positive Electron Affinity: P-type materials tend to attract electrons, which can recombine with holes, releasing energy in the process.
- Applications: P-type semiconductors are commonly used in devices such as diodes, bipolar junction transistors (BJTs), and solar cells.
Types of Semiconductor Materials: Silicon and Gallium Arsenide
Silicon and gallium arsenide represent two fundamental categories of semiconductor materials, each with distinct properties and applications. Silicon is the most widely used material in semiconductor devices due to its abundance and excellent semiconductor characteristics. It forms the basis of a vast majority of electronic devices, from microprocessors to solar cells.
Gallium arsenide, on the other hand, is known for its superior electron mobility, which allows for faster signal transmission. This makes it particularly useful in optoelectronic applications, such as LEDs and high-speed communication systems. Despite its advantages, gallium arsenide is less common than silicon because it is more expensive and complex to manufacture.
The choice between silicon and gallium arsenide in semiconductor design often comes down to a trade-off between cost and performance. Silicon’s low cost and reliability make it suitable for a wide range of applications, while gallium arsenide is preferred in specialized high-frequency and optoelectronic devices.
Types of Semiconductor Devices
Two-Terminal Devices: Diodes
Diodes are fundamental two-terminal semiconductor devices that serve as the simplest form of a p-n junction. They are designed to allow current to flow in only one direction, acting as a one-way valve for electrical current. This property makes them essential components in circuits where directionality of current is crucial.
Diodes come in various types, each tailored for specific applications. For instance, the Zener diode is widely used for voltage regulation, while the Schottky diode is known for its fast switching speed. Light-emitting diodes (LEDs) have become ubiquitous as efficient sources of light.
Here are some common types of diodes and their typical uses:
- Rectifier diode: Converts alternating current (AC) to direct current (DC)
- Zener diode: Voltage regulation
- Schottky diode: Fast switching applications
- Laser diode: Generates coherent light
- Light-emitting diode (LED): Lighting and displays
The versatility of diodes is evident in their widespread use across various electronic devices and systems.
Three-Terminal Devices: Transistors
Transistors are the fundamental building blocks for both analog and digital integrated circuits, serving as the switch or amplifier in electronic devices. Bipolar junction transistors (BJTs), which include configurations such as n-p-n or p-n-p, consist of three regions: the emitter, the collector, and the base. Field-effect transistors (FETs), on the other hand, utilize an electric field to control the conductivity and are essential in applications like 5G technology due to their efficiency.
The development of transistors has evolved significantly, with innovations such as 3D transistors enhancing performance by adding source and drain as fins of the gate. This advancement allows for higher data transfer rates and lower latency, which is crucial for next-generation wireless technology. Additionally, technologies like Through-Silicon Vias (TSVs) enable the connection of various die in a stacked configuration, further pushing the boundaries of semiconductor device capabilities.
Here is a brief comparison of the two main types of transistors:
Transistor Type | Configuration | Key Feature |
---|---|---|
BJT | n-p-n / p-n-p | Uses p-n junctions |
FET | Gate-controlled | Electric field modulation |
Transistors continue to be a subject of research and development, with emerging technologies such as Tunnel FETs poised to potentially replace current transistor models in future process technologies.
Amplifiers and Resistors
Amplifiers and resistors are fundamental components in electronic circuits, each serving a distinct purpose. Amplifiers are used to increase the power of a signal, enhancing its amplitude without altering the original signal’s information content. They are classified into various classes based on their operating characteristics, which dictate their efficiency, linearity, and heat dissipation.
Resistors, on the other hand, are passive devices that provide resistance to the flow of electric current. They are essential for controlling voltage and current within circuits, and they come in various types and sizes to suit different applications. Both amplifiers and resistors are integral to the design and function of analog circuits, oscillators, and digital logic gates.
The classification of amplifiers is an important aspect of electronics, as it determines the amplifier’s suitability for specific applications. For example, Class A amplifiers are known for high fidelity but are less efficient, while Class D amplifiers offer high efficiency but with some distortion. Understanding these classifications is crucial for selecting the right amplifier for the desired application.
Applications of Semiconductors in Technology
Microprocessors and Integrated Circuits
At the heart of modern computing lies the microprocessor, an advanced integrated circuit that embodies the functions of a computer’s CPU. Unlike simpler ICs, microprocessors execute complex instructions and manage data processing at high speeds. They are the brains within computers, smartphones, and countless other electronic devices.
Integrated circuits, on the other hand, can range from basic analog devices to complex digital systems. They integrate multiple electronic components onto a single silicon chip, enabling the miniaturization and efficiency that define contemporary electronics. The synergy between microprocessors and other ICs has led to the development of microcontrollers (MCUs), which combine processing power with memory and input/output interfaces, tailored for specific applications.
The evolution of semiconductor technology has seen a shift towards monolithic 3D chips, where transistors are stacked within a single chip, enhancing performance while reducing space. This advancement, along with the integration of analog and digital signals in mixed-signal ICs, underscores the continuous innovation in the field.
Telecommunications and Automotive Industries
In the realm of telecommunications, semiconductors are the backbone of connectivity, enabling high-speed data transmission and advanced networking capabilities. Time Sensitive Networking (TSN) is a pivotal innovation, integrating real-time communication into automotive Ethernet, which is essential for the burgeoning field of connected vehicles.
The automotive industry has witnessed a transformative impact due to semiconductors. Advanced driver-assistance systems (ADAS), infotainment, and engine control units (ECUs) are all powered by these versatile materials. The integration of semiconductors has not only enhanced vehicle performance but also significantly improved safety, marking a new height in automotive technology.
Here’s a glimpse into the various applications of semiconductors in the automotive sector:
- Advanced driver-assistance systems (ADAS): Enhancing safety and driving experience.
- Infotainment systems: Providing entertainment and vehicle information.
- Engine control units (ECUs): Optimizing engine performance and fuel efficiency.
- Sensors: Monitoring and responding to vehicle dynamics and environmental conditions.
Renewable Energy and High-Voltage Appliances
Semiconductors play a pivotal role in the renewable energy sector, particularly in the optimization of power conversion for solar panels and wind turbines. Their ability to manage high voltages and currents is essential for efficient energy transfer and storage.
In the realm of high-voltage appliances, semiconductors are integral to the design of power electronics that can withstand and regulate the substantial electrical loads. Companies like Watt & Well and Corintis are at the forefront of developing technologies that cater to these demanding applications.
- Watt & Well specializes in power electronics for mobility, energy, and manufacturing sectors.
- Corintis focuses on cooling technologies for high-performance semiconductors, crucial for maintaining system stability.
- Minima Processor’s technology significantly enhances energy efficiency in digital processors, a testament to the advancements in semiconductor applications.
P-Type Semiconductors: Characteristics and Uses
Hole Mobility and Positive Hall Coefficients
P-type semiconductors are distinguished by their positive charge carriers, known as holes, which are introduced by doping the material with elements like boron or gallium. These holes contribute to the unique electrical properties of P-type materials, including their positive Hall coefficients. The Hall coefficient is a measure of the response of a material to an applied magnetic field, and in P-type semiconductors, it indicates that holes move in the direction opposite to the magnetic field.
Hole mobility in P-type semiconductors is a critical factor that influences their performance in various applications. It is affected by several parameters:
- Temperature: Higher temperatures can increase the mobility of holes.
- Impurity concentration: The presence of dopants can either enhance or hinder hole mobility.
- Crystal structure: The arrangement of atoms in the semiconductor affects how easily holes can move.
Despite having lower electron mobility compared to N-type semiconductors, P-type materials are essential in the production of devices like solar cells and diodes due to their ability to efficiently transport positive charge.
Applications in LEDs, BJTs, and Solar Cells
P-type semiconductors are integral to the functionality of various electronic components. LEDs, or light-emitting diodes, rely on the properties of P-type materials to form p-n junctions, which are essential for their ability to emit light when current is applied. These semiconductors are doped with elements from Group III of the periodic table to achieve the desired characteristics.
In the realm of solar energy, P-type semiconductors are indispensable. They are used in solar cells to absorb photons from sunlight, creating electron-hole pairs that are critical in the generation of electric current. This process is vital for converting solar energy into usable electricity, making P-type materials a cornerstone in the field of renewable energy.
Moreover, P-type semiconductors find their use in bipolar junction transistors (BJTs), which are a type of transistor that uses both electron and hole charge carriers. Their unique hole mobility characteristics make them suitable for amplifying current, and they are commonly used in various electronic circuits and devices.
Thermoelectric Effects and MEMS Production
P-type semiconductors are pivotal in the realm of thermoelectric effects, where they are harnessed to convert temperature differentials directly into electrical voltage. This phenomenon, known as the Seebeck effect, is integral to thermal sensing and energy harvesting applications. The efficiency of p-type semiconductors in these applications is often characterized by the Seebeck coefficient, a parameter that quantifies the induced voltage per unit temperature gradient.
In the manufacturing landscape, p-type semiconductors find extensive use in the production of Microelectromechanical Systems (MEMS). These tiny yet complex devices combine mechanical and electrical components at a microscale, leading to innovations in sensors, actuators, and other miniature systems. The integration of p-type semiconductors in MEMS technology has been a driving force behind their miniaturization and the enhancement of their functional capabilities.
The following table summarizes the roles of p-type semiconductors in thermoelectric effects and MEMS production:
Application Area | Role of P-Type Semiconductors |
---|---|
Thermoelectric Devices | Harvesting waste heat to generate electricity |
MEMS Production | Enabling miniaturization and functionality of components |
Advancements and Manufacturing Techniques
Lithography, Etching, and Deposition Processes
The fabrication of semiconductor devices relies heavily on the precision and control offered by lithography, etching, and deposition processes. Lithography serves as the cornerstone, using light to transfer patterns from a photomask onto a substrate. This intricate process often involves complex coefficients, such as the lithography K1 coefficient, which indicates the difficulty of the process.
Following lithography, etching techniques like Atomic Layer Etch (ALE) are employed to selectively remove materials at the atomic scale, ensuring the fidelity of the patterned features. ALE represents a significant advancement in the etching domain, allowing for unprecedented precision.
Deposition processes, including Atomic Layer Deposition (ALD), play a crucial role in adding materials to the substrate with atomic accuracy. These methods, such as metal-organic chemical vapor deposition (MOCVD), enable the construction of layers necessary for the device’s functionality. The table below summarizes the key processes and their respective roles in semiconductor manufacturing:
Process | Role in Manufacturing | Example Technologies |
---|---|---|
Lithography | Pattern Transfer | Photomask, Double Patterning |
Etching | Material Removal | ALE, Plasma-Enhanced Etching |
Deposition | Material Addition | ALD, MOCVD |
The continuous evolution of these techniques, including the development of new methods like roll-to-roll and plasma-enhanced deposition, is pivotal to the future of semiconductor device manufacturing.
Nanotechnology in Semiconductor Fabrication
The integration of nanotechnology in semiconductor fabrication marks a significant leap forward in the miniaturization and performance of electronic devices. This approach utilizes the manipulation of materials at the atomic or molecular level to create structures that are often only a few nanometers in size. The precision afforded by nanotechnology allows for the development of semiconductor components with enhanced electrical properties and reduced power consumption.
Recent collaborations and investments in nanofabrication equipment, such as the new set provided by Applied Materials to MIT.nano, are indicative of the industry’s commitment to pushing the boundaries of semiconductor technology. These advanced tools are capable of fabricating wafers, which are essential for the production of microchips with increasingly complex and integrated circuits.
The potential applications of nanotechnology in semiconductor fabrication are vast, ranging from the creation of more efficient memory devices to the development of microelectromechanical systems (MEMS). As the industry continues to innovate, the strategic focus on reshoring microchip production in the United States underscores the importance of maintaining a robust research infrastructure for these technological advancements.
Future Trends in Semiconductor Technology
The semiconductor industry is on the brink of transformative changes, with emerging trends poised to redefine the landscape of chip manufacturing and design. The 2024 Semiconductor Industry Outlook by Deloitte highlights several trends that are expected to shape the market in the coming year. These include the integration of artificial intelligence in semiconductor processes, the adoption of advanced materials like graphene, and the shift towards domain-specific accelerators to meet the demands of AI and machine learning workloads.
In response to these trends, companies are exploring novel manufacturing techniques, such as the use of ‘donut’ beams and ‘Lego’ block approaches, to overcome the limitations of traditional lithography. The push for innovation is also evident in the research on silicon photonics, which aims to achieve computing at the speed of light, revolutionizing data transfer and processing.
As geopolitical tensions influence the semiconductor supply chain, nations are taking strategic steps to secure their chip industries. South Korea’s implementation of its own Chips Act and Intel’s announcement of a new Bitcoin mining chip are indicative of the dynamic nature of the industry. With the global semiconductor market projected to reach $1.41 trillion by 2032, the future holds exciting prospects for advancements that will continue to revolutionize industries and enhance our daily experiences.
Conclusion
In summary, semiconductors are the cornerstone of modern electronics, bridging the gap between conductors and insulators with their unique ability to control conductivity. This article has explored the various types of semiconductor materials, their distinctive properties, and their widespread applications, from microprocessors to high-voltage appliances. We’ve delved into the intricacies of two-terminal and three-terminal devices, such as diodes and transistors, and highlighted the significance of P-type semiconductors in technology like LEDs, ICs, and MEMS. As we’ve seen, the role of semiconductors extends far beyond these examples, influencing industries such as telecommunications, automotive, and renewable energy. With ongoing advancements in semiconductor technology, including nanotechnology and new manufacturing techniques, the potential for innovation is boundless. Understanding semiconductors is not just about comprehending materials and devices; it’s about envisioning the future of technological progress.
Frequently Asked Questions
What are the different types of semiconductor materials?
The most common semiconductor materials are silicon and gallium arsenide, each with unique properties that make them suitable for various applications.
What are the two types of semiconductor devices?
Semiconductor devices can be categorized into two-terminal devices, such as diodes, and three-terminal devices, such as transistors.
What is a common application of semiconductor materials?
Semiconductor materials are commonly used in the creation of microprocessors and are also suitable for high-voltage appliances.
How are semiconductor devices used in technology?
Semiconductor devices are used in digital circuits, microprocessors, oscillators, amplifiers, and high-voltage applications, among others.
What role do P-type semiconductors play in technology?
P-type semiconductors are essential for creating devices like light-emitting diodes (LEDs), bipolar junction transistors (BJTs), and solar cells, as well as in the production of microelectromechanical systems (MEMS).
What are some examples of semiconductor devices?
Examples of semiconductor devices include operating-amplifiers, resistors, and integrated circuits, which are integral to electronic equipment.