Navigating the Complexities of Modern IC Circuit Design
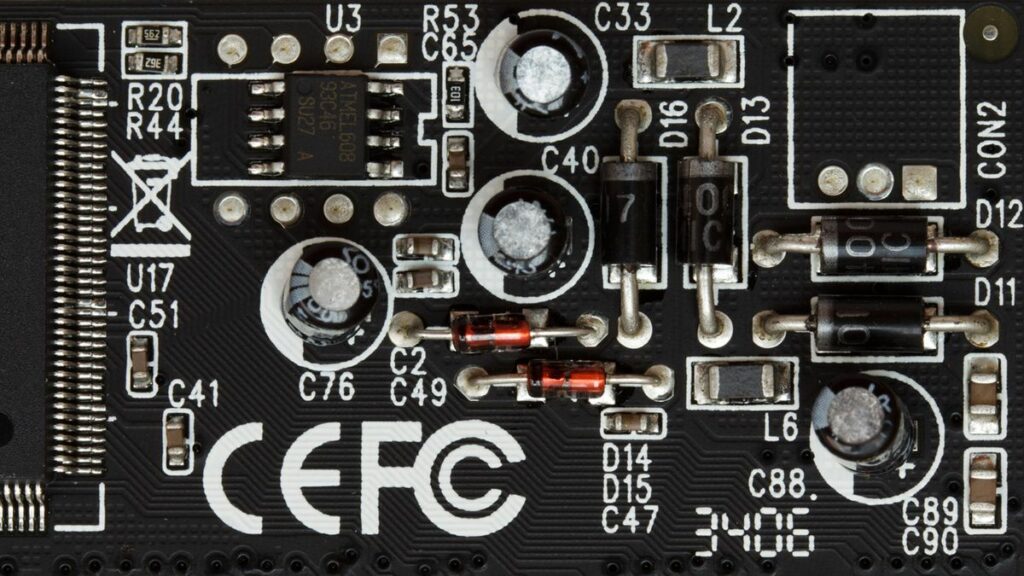
Navigating the complexities of modern Integrated Circuit (IC) design requires a deep understanding of the evolutionary trends, design methodologies, and environmental and operational constraints that shape today’s semiconductor industry. From the shift towards multi-chiplet architectures and fabless manufacturing to the integration of advanced packaging and ensuring IC integrity, each aspect plays a pivotal role in the development of robust and efficient electronic systems. This article delves into these intricate aspects, providing insights into the state-of-the-art tools and strategies that address the challenges of RF PCB design, optimize performance, and maintain the foundation of trust in computing systems.
Key Takeaways
- The transition from single-chip to multi-chiplet architectures and the move to fabless manufacturing have revolutionized IC design, necessitating advanced packaging techniques for optimal system performance.
- Modern design tools like Cadence’s complete design flow with EM and Thermal Analysis are critical for addressing the increasing complexity of IC designs, especially for EM and thermal management in 3D heterogeneous solutions.
- Designing for extreme environmental conditions and ensuring reliability beyond standard PDK ranges require collaborative efforts and a holistic approach that merges diverse design expertise.
- IC integrity and security are paramount, with techniques like reverse engineering, logic locking, and SAT attack resistance being essential to safeguard the trust in computing systems.
- Balancing minimal routing with performance needs, employing cost-effective design strategies, and streamlining the transition to heterogeneous integrated solutions are key for optimizing IC performance, price, and manufacturability.
The Evolution of IC Circuit Design in the Modern Era
From Single Chips to Multi-Chiplet Architectures
The transition from single-chip systems to multi-chiplet architectures represents a paradigm shift in IC circuit design. This modular approach leverages the concept of ‘chiplets’, which are smaller, function-specific chips that can be combined to form a larger system. By breaking down a system into chiplets, designers can achieve better yields and lower costs, as smaller chips have a lower probability of manufacturing defects. Moreover, this strategy allows for the customization of process technology for each chiplet, optimizing the use of advanced nodes where necessary and employing less expensive technologies for other components.
The benefits of chiplet-based designs extend to upgradeability as well. Designers can update or enhance specific functional blocks without the need to overhaul the entire system. This flexibility is crucial in the fast-paced world of technology where rapid iteration and improvement are necessary. The table below summarizes the key advantages of adopting multi-chiplet architectures:
Advantage | Description |
---|---|
Better Yields | Smaller chips result in a lower likelihood of defects. |
Cost Efficiency | Custom process technology for each chiplet reduces overall costs. |
Upgradeability | Individual chiplets can be updated without redesigning the whole system. |
As the computational demands of applications like AI supercomputers grow, the spotlight has intensified on Systems in Package (SiPs) and their ability to expand beyond traditional size limits. The design of chiplets involves considerations such as packaging, high-speed chip-to-chip interconnects, and the interoperability and standardization necessary for combining chips from different vendors.
The Shift to Fabless Manufacturing and Global Outsourcing
The semiconductor industry has undergone a significant transformation with the shift to fabless manufacturing and global outsourcing. This model allows companies to focus on the design and sale of semiconductors while entrusting the production to specialized foundries. The benefits of this approach include reduced capital expenditure and the ability to leverage the expertise and economies of scale of established manufacturers.
However, this shift also introduces new challenges. Companies must navigate complex relationships with multiple vendors, often across different countries, and manage the risks associated with intellectual property protection and supply chain disruptions. The table below highlights some of the key collaborations and events in the industry:
Date | Event | Description |
---|---|---|
Feb 26, 2024 | Cadence and Intel Foundry collaborate | A partnership to enhance IC design capabilities. |
Feb 23, 2024 | Infineon sells manufacturing sites | A strategic move to streamline operations. |
Feb 23, 2024 | Renesas develops AI accelerator | Advancement in AI technology for ICs. |
To mitigate risks such as unauthorized overproduction and intellectual property theft, companies are implementing security measures like logic locks, which ensure that a chip’s functionality is accessible only with a specific key known to the designer.
The Role of Advanced Packaging in System Performance
Advanced packaging technologies have become a cornerstone in enhancing the performance of modern integrated circuits (ICs). The integration of multiple chiplets into a single package has necessitated the development of sophisticated packaging flows. For instance, Cadence’s Allegro X APD and Integrity 3D-IC Platform offer tools for placement, routing, and electrical analysis, which are critical for ensuring signal integrity and reducing insertion losses.
The collaboration between Cadence and Intel Foundry exemplifies the industry’s push towards optimizing packaging for high-performance computing (HPC), AI, and mobile computing. The use of Embedded Multi-die Interconnect Bridge (EMIB) technology is a testament to the evolving landscape of IC design, where cost and power efficiency are balanced against data rate and transmission distance capabilities.
Key aspects of advanced packaging impacting system performance include:
- Signal integrity and power analysis
- Co-engineering across disciplines
- Integration of mm-wave and sub-THz packaging
- Cost-effective module realization
These elements are crucial for addressing the challenges posed by the latest generation of ICs, ensuring that the packaging not only meets the technical requirements but also aligns with the economic constraints of production.
Design Methodologies and Tools for Next-Generation ICs
Cadence’s Complete Design Flow for EM and Thermal Analysis
Cadence’s suite of tools for electromagnetic (EM) and thermal analysis is integral to modern IC design, addressing the complexities that arise from the need to optimize system performance. The design flow includes a variety of specialized software solutions, each tailored to specific aspects of the design process. For instance, the Clarity 3D Solver and Celsius solvers are pivotal for EM extraction and thermal signoff, respectively, ensuring that designs meet stringent performance criteria from the earliest stages.
The comprehensive design flow also encompasses tools for signal integrity, power analysis, and packaging model extraction. This allows for a holistic approach to design, where all critical factors are considered in unison. The following list highlights key components of Cadence’s design flow:
- Sigrity and Clarity solvers for 3D EM extraction and signal integrity analysis
- Celsius solvers for thermal analysis and stress testing
- Virtuoso Studio for routing of EMIB bridges
- Pegasus Verification System for signoff DRC and SystemLVS
By integrating these tools into a unified workflow, designers can navigate the intricate balance between performance, price, and manufacturability, ultimately leading to more efficient and reliable IC products.
Integrating 3D Heterogeneous Solutions with EDA Tools
The integration of 3D heterogeneous solutions with Electronic Design Automation (EDA) tools is a pivotal advancement in IC circuit design. Now, designers can anticipate and resolve potential performance issues before manufacturing, such as the impact of chip placement within a package or the proximity of multiple chips to one another. This foresight is crucial in avoiding costly design iterations and ensuring optimal performance.
Key EDA tools have been enhanced to support 3D heterogeneous integration, offering capabilities that span from system-level design to detailed electrical analysis. For instance, tools like Cadence’s Allegro X APD and Integrity 3D-IC Platform facilitate advanced packaging flow, including placement, routing, and analysis. The integration with solvers such as Sigrity and Clarity further enables comprehensive signal integrity and power analysis.
The table below outlines the key features of EDA tools that support 3D heterogeneous integration:
Feature | Description |
---|---|
Placement & Routing | Facilitates signal/power/ground routing and manufacturing output. |
Electrical Analysis | In-design analysis for signal integrity and power distribution. |
System-Level Planning | Enables design aggregation, planning, and optimization. |
EM Extraction | Provides 3D electromagnetic extraction and model generation. |
By leveraging these tools, designers can collaborate more effectively, addressing complex design challenges with a higher level of precision and confidence. The result is a streamlined transition to heterogeneous integrated solutions, balancing performance with manufacturability.
Addressing the Challenges of RF PCB Design
Radio frequency (RF) printed circuit board (PCB) design is a critical component in the realm of wireless communication systems, where precision and performance are paramount. Designing RF PCBs requires a deep understanding of high-frequency signal behaviors to ensure signal integrity and minimize interference. Material selection, stackup configuration, and signal routing are just a few of the many factors that must be meticulously managed.
To address these challenges, engineers employ a variety of strategies:
- Utilizing materials with appropriate dielectric properties to maintain signal integrity.
- Designing stackups that mitigate cross-talk and electromagnetic interference (EMI).
- Implementing controlled impedance lines to ensure consistent signal transmission.
These strategies are underpinned by adherence to industry standards and a thorough thermal management plan to prevent performance degradation. The complexity of RF PCB design necessitates the use of specialized tools and advanced design techniques to meet the high standards required for today’s wireless communication systems.
Overcoming Environmental and Operational Constraints
Designing for Extreme Environmental Conditions
Designing integrated circuits to withstand extreme environmental conditions is a formidable challenge that necessitates a blend of specialized design skills. Emerging applications, such as satellite-based internet and high-temperature industrial sensors, demand ICs that function reliably across a broad spectrum of temperatures and conditions. For instance, circuits operating in space must endure the vacuum of space and radiation, while those used in deep-sea exploration face immense pressure and corrosive elements.
To address these demands, designers must extend the capabilities of standard Process Development Kits (PDKs) and innovate with materials and architectures that can tolerate such extremes. For example, cryogenic CMOS design requires an understanding of device behavior at temperatures near zero Kelvin, while high-temperature electronics must maintain performance above 150C. The table below summarizes some of the environmental conditions and the corresponding design considerations:
Environment | Temperature Range | Design Considerations |
---|---|---|
Cryogenic | Near 0K – 150K | Device behavior, matching |
High Temp | Above 150C | Yield, material stability |
Radiation | Various levels | Circuit hardening, redundancy |
Collaboration across different design communities is essential to overcome these challenges. Workshops and conferences that bring together experts from academia and industry play a crucial role in sharing knowledge and developing holistic strategies for designing ICs that can operate in the most demanding environments.
Ensuring Reliability Beyond Standard PDK Ranges
The relentless pursuit of innovation in IC design often pushes the boundaries of standard Process Design Kits (PDKs). To ensure reliability beyond these ranges, engineers are turning to custom solutions that integrate seamlessly with existing design tools. These bespoke systems are tailored to address specialized use cases where out-of-the-box options fall short, and they often involve a hybrid model of a core platform augmented with targeted integrations.
A proactive approach to design verification is crucial. By employing a multifaceted strategy that includes directed, random, and assertion-based testing, engineers can validate IC functionality and performance while ensuring compliance with industry standards. This comprehensive testing is especially important in fields like RF PCB design, where maintaining standards for wireless communication is paramount.
To support these efforts, a Chip Design Hub should provide access to a variety of PDK and IP resources, broadening participation in IC chip design. The table below outlines key aspects of ensuring reliability in IC design:
Aspect | Description |
---|---|
Custom Solutions | Tailored systems with SDK integration for specialized needs. |
Design Verification | Combining testing techniques for robust IC designs. |
Resource Access | Providing diverse PDK and IP resources via a Chip Design Hub. |
Collaborative Approaches to Complex Design Challenges
In the realm of modern IC circuit design, collaboration is pivotal. Engineers from various disciplines—electrical, mechanical, layout, and verification—must have shared access to design data to exchange insights and drive refinements. This necessitates a robust data management system that not only facilitates this exchange but also safeguards intellectual property.
The implementation of PCB data management systems is guided by evaluating core motivations and balancing them against the costs and challenges of adopting new platforms. A successful data management strategy can lead to significant improvements in security, quality, accessibility, and engineering productivity. Below is a list of key considerations for effective data management rollout:
- Evaluating organizational needs against specific tool gaps
- Ensuring IP protection with secure data distribution channels
- Centralizing and standardizing data to streamline collaboration
- Overcoming IT resource constraints and change adoption hurdles
Ultimately, a unified platform that bridges remote teams can lead to hard cost reductions from fewer change orders and soft benefits such as increased staff efficiency. These factors make investments in PCB data management systems not only necessary but also financially beneficial in the long run.
Ensuring IC Integrity and Security
Reverse Engineering and Decamouflaging Techniques
The practice of reverse engineering is pivotal in understanding and analyzing the design and functionality of integrated circuits (ICs). It involves deconstructing an IC to reveal its architecture, components, and underlying logic. This process is not only used for legitimate purposes such as learning and innovation but also poses a significant threat to intellectual property and security when used maliciously.
Decamouflaging, on the other hand, specifically targets ICs designed with obfuscation techniques to protect proprietary designs. Researchers have developed various methods to decamouflage and analyze such protected circuits. Below is a list of notable publications that have contributed to the field:
- M. El Massad et al., NDSS (2015): Integrated circuit (IC) decamouflaging within minutes.
- M. Yasin et al., HOST Symposium (2016): SAT attack resistant logic locking.
- B. Shakya et al., CHES (2019): Protecting ICs with undetectable camouflaging.
- T. Meade et al., ISCAS (2017): Revisiting sequential logic obfuscation.
These studies highlight the ongoing arms race between circuit designers seeking to protect their work and adversaries aiming to uncover protected designs. The implications of these techniques extend beyond academic interest, affecting the trust and security of computing systems worldwide.
Protecting Against SAT Attacks with Logic Locking
The threat of SAT (Satisfiability) attacks on logic-locked ICs has prompted the development of more robust countermeasures to ensure IP security. Provably-secure logic locking mechanisms have emerged as a critical defense, effectively thwarting attempts to reverse-engineer the circuit’s functionality. Researchers have proposed various enhancements to traditional logic locking, such as Anti-SAT and cyclic obfuscation, which aim to create SAT-unresolvable circuits.
Recent studies have highlighted the effectiveness of these techniques:
- Y. Xie and A. Srivastava demonstrated the mitigation of SAT attacks through improved logic locking (CHES 2016).
- M. Yasin et al. presented a provably-secure logic locking method that balances security and practicality (CCS 2017).
- Y. Liu et al. introduced Strong Anti-SAT, a secure and effective logic locking approach (ISQED 2020).
These advancements underscore the importance of continuous innovation in logic locking to protect against evolving SAT attacks. The resilience of logic-locked circuits is paramount for maintaining the foundation of trust in computing systems, as they safeguard against unauthorized access and tampering with the IP.
Maintaining the Foundation of Trust in Computing Systems
In the realm of integrated circuit (IC) design, maintaining trust throughout the manufacturing process and lifecycle of the chip is paramount. Ensuring the integrity and security of ICs is a multifaceted challenge that involves protecting against various forms of attacks and unauthorized access. Recent research has focused on developing robust design-for-security architectures and methodologies that can be integrated into the IC design flow.
One such approach is the implementation of logic locking techniques, which serve to protect against reverse engineering and Intellectual Property (IP) theft. These techniques involve the insertion of additional gates or circuits that require a specific key to unlock the full functionality of the IC. The table below summarizes some of the key techniques and their references:
Technique | Description | Reference |
---|---|---|
Design-for-Security Architecture | Enhances trust in IC manufacturing and test | [53] |
Keygate Placement and Dynamic Scan Obfuscation | Protects against robust logic encryption | [54] |
Delay Locking | Secures logic locking against counterfeiting | [3] |
High-Level Language Locking (HLock) | Locks IPs at the high-level language to prevent tampering | [32] |
Collaboration between academia and industry is crucial to advance these security measures. Initiatives like DARPA’s program to increase semiconductor supply chain security highlight the ongoing efforts to establish a foundation of trust in computing systems. As threats evolve, so must the strategies to mitigate them, ensuring that ICs remain secure throughout their lifecycle.
Optimizing Performance, Price, and Manufacturability
Balancing Minimal Routing with Performance Needs
In the realm of IC circuit design, the quest for minimal routing is often at odds with the need for high performance. Signal routing is not just about connecting points A to B; it’s about doing so in a way that preserves signal integrity and meets stringent performance criteria. Employing controlled impedance traces and differential pair routing techniques are essential for reducing signal reflections, attenuations, and electromagnetic interference, which are critical for high-frequency signal transmission.
To achieve this balance, designers must consider a variety of factors. Material selection, impedance control, and routing optimization are just a few of the elements that must be harmonized to ensure optimal performance and reliability. The table below summarizes key considerations for minimal routing that does not compromise performance:
Factor | Consideration | Impact |
---|---|---|
Impedance Control | Tailoring to specific signal frequencies | Minimizes signal reflections |
Routing Techniques | Utilizing differential pairs | Reduces EMI and crosstalk |
Material Selection | Choosing appropriate substrates | Affects signal integrity |
As the complexity of designs escalates, tools like Cadence’s complete design flow for EM and Thermal Analysis become indispensable. These tools help designers navigate the intricate trade-offs between minimal routing, performance, and manufacturability, ensuring that the final product functions with optimal performance and reliability.
Cost-Effective Design Strategies for IC Production
In the pursuit of cost-effective design strategies for IC production, designers are increasingly looking at ways to optimize the manufacturability of their designs while keeping costs low. Downsizing boards as much as the circuit allows or utilizing multiple tiny boards for different functions can lead to significant savings, especially for prototypes or low volume runs. This approach not only reduces material costs but also minimizes the risk of defects, enhancing overall yield.
Adopting a multi-chiplet architecture allows for the segregation of large complex systems into smaller, function-specific chips. This not only improves yields due to a lower probability of manufacturing defects but also enables cost reductions by tailoring the process technology to each chiplet. For instance, advanced nodes can be reserved for high-performance components like GPUs and CPUs, while less expensive technologies can be employed for memories and analog interfaces. Moreover, this modular approach facilitates targeted upgrades to certain functional blocks without necessitating a complete system redesign.
The table below outlines key considerations for cost-effective IC design:
Factor | Description |
---|---|
Board Downsizing | Minimize board size to reduce material costs. |
Multi-Chiplet Architecture | Use smaller, specialized chips to improve yields and lower costs. |
Process Customization | Select appropriate technology nodes for different chiplets. |
Design Upgradability | Enable updates to specific blocks without full system redesign. |
By integrating these strategies, designers can achieve a delicate balance between performance, price, and manufacturability, ensuring that the transition to heterogeneous integrated solutions is both efficient and cost-effective.
Streamlining the Transition to Heterogeneous Integrated Solutions
The transition to heterogeneous integrated solutions is a pivotal moment in IC design, where the integration of various functional components such as logic chips, sensors, and memory is essential. This integration not only aims for higher interconnection density but also encapsulates the complexity of combining disparate technologies into a cohesive system. Advanced packaging design, like the ASE’s approach to 3D Heterogeneous Integration (3DHI), is critical in this respect, enabling the stacking of separately manufactured components to create a unified architecture.
To facilitate this shift, industry collaborations are proving invaluable. For instance, the partnership between Cadence and Intel Foundry has led to the development of an integrated advanced packaging flow using Embedded Multi-die Interconnect Bridge (EMIB) technology. This collaboration is tailored to meet the demands of high-performance computing (HPC), AI, and mobile computing, allowing design teams to seamlessly transition to advanced multi-chip(let) architectures.
Moreover, the standardization of die-to-die (D2D) interfaces is a significant step towards leveraging chiplet-based architectures. It ensures interoperability and allows developers to engage with multiple vendors, enhancing flexibility and scalability. Custom solutions, such as bespoke systems built on databases and SDK integration, address specialized use cases by tapping into native design tool capabilities, where out-of-box options fall short.
Conclusion
In conclusion, the intricacies of modern IC circuit design are vast and multifaceted, encompassing a range of considerations from simulation and verification processes like DRC, LVS, ERC, EM, and PI, to challenges in thermal management, minimal routing, and system performance. The shift towards fabless manufacturing, multi-chiplet architectures, and advanced packaging further complicates the design landscape, necessitating robust design tools and methodologies. Cadence’s comprehensive design flow, including EM and Thermal Analysis, provides a critical infrastructure for addressing these complexities and is instrumental in the development of next-generation designs. As we continue to push the boundaries of technology, it is imperative for designers to integrate their work within the larger system context early in the design flow and to utilize advanced EDA tools to predict and mitigate potential issues before manufacturing. The collaboration of industry and academia, as well as the sharing of expertise across traditionally separate design communities, will be key to overcoming the challenges and ensuring the reliability and performance of ICs in the demanding environments of tomorrow’s electronic systems.
Frequently Asked Questions
How has IC circuit design evolved in the modern era?
IC circuit design has progressed from single-chip solutions to complex multi-chiplet architectures, leveraging advanced packaging for improved system performance. Fabless manufacturing and global outsourcing have become prevalent as feature sizes shrink, requiring sophisticated design methodologies and tools.
What role does Cadence’s design flow play in IC design?
Cadence’s complete design flow is essential for addressing electromagnetic (EM), power integrity (PI), and thermal analysis challenges in next-generation IC designs. It serves as the backbone infrastructure, integrating with EDA tools to facilitate 3D heterogeneous solutions and streamline designs earlier in the flow for optimal system performance.
What are the challenges in RF PCB design?
RF PCB design challenges include maintaining signal integrity, controlling impedance, and mitigating electromagnetic interference in high-frequency environments. Designers must use advanced techniques and specialized tools to ensure that RF PCBs meet the demanding performance criteria of modern wireless technologies.
How do environmental conditions affect IC design?
ICs must be designed to operate in extreme environmental conditions, often beyond the standard ranges provided by Process Development Kits (PDK). Collaborative approaches and expertise from various design communities are necessary to overcome these challenges and ensure reliability across diverse operating voltages and temperatures.
What measures are taken to ensure IC integrity and security?
To ensure IC integrity and security, techniques such as reverse engineering, decamouflaging, and logic locking (e.g., SARLock) are employed to protect against threats like SAT attacks. These measures maintain the foundation of trust in computing systems by preventing unauthorized access and tampering.
How can performance, price, and manufacturability be optimized in IC design?
Optimizing IC design involves balancing performance needs with minimal routing, employing cost-effective design strategies, and streamlining the transition to heterogeneous integrated solutions. This requires careful consideration of manufacturability and market price while ensuring the desired performance levels are met.