The Evolution of Semi-Conductors: An In-Depth Exploration
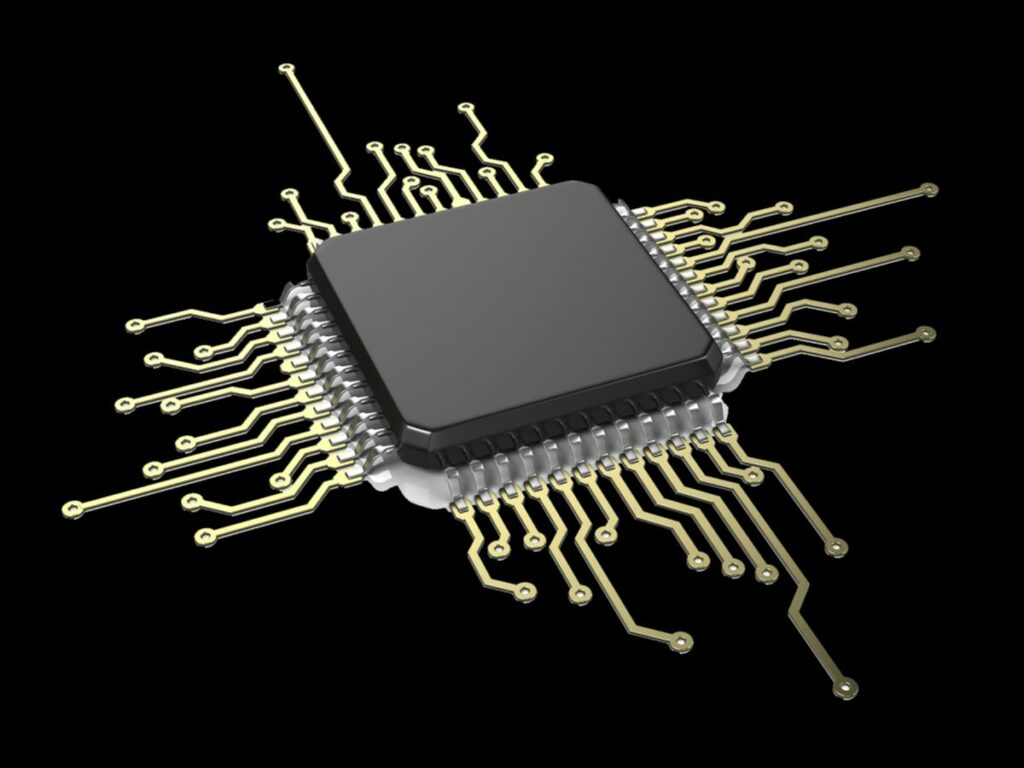
The history of the microchip is a tale of human ingenuity, relentless innovation, and a quest to push the boundaries of what’s possible. This article takes readers on a detailed journey through the evolution of semiconductors, from the early days of the transistor to the cutting-edge technologies of the 2020s. We’ll explore key developments, the impact of Moore’s Law, the introduction of new materials, and the future of microelectronics.
Key Takeaways
- The invention of the transistor marked the beginning of the microelectronics era, revolutionizing the way electronic devices are built.
- Moore’s Law has been a guiding principle in the semiconductor industry, predicting the exponential growth of integrated circuit complexity.
- Advancements in materials and manufacturing techniques have continuously improved semiconductor performance and efficiency.
- The integration of AI and machine learning into chip design is shaping the future of microchip technology, making it more adaptive and powerful.
- Nanotechnology and the quest for quantum computing are paving the way for the next generation of breakthroughs in semiconductor technology.
The Dawn of Microelectronics: Laying the Foundation
The Birth of the Transistor
The invention of the transistor in 1947 by John Bardeen, Walter Brattain, and William Shockley marked a pivotal moment in the history of technology. Developed at Bell Labs, this groundbreaking device was capable of amplifying and switching electronic signals, heralding a new era in electronics. The transistor was a quantum leap forward from the bulky and inefficient vacuum tubes of the time, offering a path to miniaturization and reliability that would shape the future of electronic devices.
The significance of the transistor extends beyond its immediate applications. It laid the groundwork for the integrated circuits that would come to dominate the microelectronics industry. The ability to control electron flow through a crystal was a transformative discovery, one that would lead to the development of smaller, faster, and more energy-efficient components.
Here is a brief timeline highlighting the key milestones following the invention of the transistor:
- 1947: Invention of the first transistor at Bell Labs.
- 1950s: Transistors begin to replace vacuum tubes in electronic devices.
- 1960s: The first integrated circuits emerge, combining multiple transistors on a single chip.
The transistor’s journey from a laboratory breakthrough to a cornerstone of modern electronics is a testament to the ingenuity and foresight of its inventors. It set the stage for the digital age, where transistors are now ubiquitous, found in everything from smartphones to spacecraft.
From Vacuum Tubes to Silicon Chips
The transition from vacuum tubes to silicon chips marked a pivotal moment in the history of electronics. Transistors emerged as a superior alternative, offering a range of benefits over their vacuum tube predecessors. They were smaller, more reliable, and consumed less power, which allowed for the miniaturization of electronic devices and paved the way for the modern computing era.
The advantages of transistors over vacuum tubes can be summarized as follows:
- Less expensive: Transistors became more cost-effective to produce.
- Smaller size: Their compact nature enabled the development of smaller devices.
- Increased reliability: Transistors did not burn out as vacuum tubes did, leading to longer-lasting electronics.
- Lower power consumption: This characteristic was crucial for battery-powered devices.
Engineers quickly adapted the principles of transistor technology to create other components like resistors and capacitors. This innovation reduced the need for cumbersome wiring and allowed for the creation of integrated circuits, which further compacted the complexity of electronic systems into tiny chips.
The Rise of Semiconductor Giants
The 1960s marked a significant turning point in the semiconductor industry with the emergence of companies that would become titans of technology. Fairchild Semiconductor and Intel, spearheaded by visionaries like Robert Noyce and Gordon Moore, were at the forefront of innovation in microchip technology. Their efforts were instrumental in shrinking the size and cost of electronic components, democratizing access to technology and setting the stage for an explosion of electronic devices across various sectors.
The process of semiconductor device fabrication has become a complex and specialized field, involving a multitude of companies. Notably, firms such as ASML, Applied Materials, Tokyo Electron, and Lam Research play critical roles in manufacturing the sophisticated machinery required for the industrial fabrication of semiconductors.
As the industry expanded, the 1970s and beyond saw the mass production and globalization of microchip manufacturing. Technological advancements in photolithography and silicon wafer fabrication allowed for the production of microchips on an unprecedented scale, reducing costs and facilitating the spread of technology worldwide. The rise of Silicon Valley epitomized the tech revolution, becoming a magnet for talent and investment globally.
The Era of Microprocessors: The Age of Proliferation
The Microprocessor Revolution
The microprocessor revolution marked a pivotal shift in the landscape of computing and electronics. In 1971, Intel’s introduction of the 4004 chip, the world’s first commercially available microprocessor, signaled the dawn of the personal computing era. This compact yet powerful chip could perform the functions of a computer’s central processing unit, setting the stage for the development of the first personal computers.
These early computers, such as the Altair 8800 and the Apple I, though primitive by today’s standards, were transformative. They brought computing power to individuals and small businesses, democratizing access to technology. The microprocessor’s impact was profound, enabling not just personal computing, but also advancing the capabilities of electronic devices across various industries.
The table below highlights the rapid progression of microprocessor technology through the decades:
Year | Milestone Microprocessor | Notable Feature |
---|---|---|
1971 | Intel 4004 | First commercial microprocessor |
1974 | Intel 8080 | Enabled the creation of the first PCs |
1981 | IBM PC’s Intel 8088 | Popularized personal computing |
1993 | Intel Pentium | Introduced superscalar architecture |
The adoption of integrated circuits (ICs) in high-profile applications like NASA’s Apollo Guidance Computer during the 1960s underscored the reliability and potential of microchip technology. By the end of the decade, the integrated circuit had become a fundamental building block of modern electronics, paving the way for the microprocessor revolution that would follow.
The Impact of Moore’s Law
The influence of Moore’s Law on the electronics industry is undeniable. Initially observed by Gordon Moore in 1965, it has become a benchmark for technological progress, suggesting that the number of transistors on a microchip doubles approximately every two years. This has led to the expectation of rapid, continual improvement in electronic devices, compelling companies to innovate constantly.
However, as we approach the physical limits of transistor miniaturization, the industry faces challenges in sustaining this pace. The focus has shifted from merely shrinking components to enhancing efficiency, discovering new materials, and considering alternative computing models like quantum computing. Despite these hurdles, the essence of Moore’s Law continues to drive the industry forward, embodying the relentless human pursuit of innovation.
The following table illustrates the exponential growth in computing power over the decades, as predicted by Moore’s Law:
Year | Transistor Count (approximate) |
---|---|
1971 | 2,300 |
1981 | 120,000 |
1991 | 1,000,000 |
2001 | 42,000,000 |
2011 | 2,600,000,000 |
2021 | 50,000,000,000 |
This trend has had profound implications, transforming a simple observation into a driving force for the entire electronics sector. The smartphone in your pocket today is a testament to this, being exponentially more powerful than the computers used for the Apollo moon landings.
Mass Production and Global Expansion
The latter part of the 20th century marked a significant shift in the semiconductor industry with the onset of mass production and the globalization of microchip manufacturing. Advances in photolithography and silicon wafer fabrication were pivotal, enabling the production of microchips on an unprecedented scale while reducing costs. This democratization of technology spurred innovation and competition across the globe.
The rise of Silicon Valley became a cultural and economic phenomenon, emblematic of the tech revolution. It drew talent and investment from every corner of the world, establishing a new epicenter for technological advancement. The impact of this growth extended far beyond the borders of the United States, with companies like Taiwan’s TSMC leading the charge in global expansion, as evidenced by their recent opening of a plant in Japan.
Microchips have revolutionized industries by automating manufacturing processes, enhancing efficiency and precision. This transformation is evident in sectors as diverse as automotive and consumer goods. The following list highlights the key areas impacted by the proliferation of microchips:
- Driving the Digital Revolution: The backbone of modern computing and digital services.
- Advancements in Communication: Enabling rapid and global connectivity.
- Revolutionizing Industries: Automation and innovation in various sectors.
- Enhancing Everyday Life: From smartphones to smart homes.
- Economic Impact: Significant contributions to global economies.
- Research and Development: Fueling technological breakthroughs.
- Environmental and Societal Impact: Shaping sustainable and societal changes.
- Defense and Security: Critical to national defense systems.
Advancements and Innovations: Pushing the Boundaries
The Introduction of New Semiconductor Materials
The relentless pursuit of enhanced performance and efficiency in the semiconductor industry has led to the introduction of new materials that are pivotal in the evolution of microelectronics. High-k dielectrics and metal gates have emerged as game-changers, significantly reducing leakage current and boosting chip performance to meet the demands of advanced computing.
The process of creating semiconductor materials often begins with silicon, sourced from sand rich in silicon dioxide. This silicon must undergo a rigorous purification process, ensuring the highest quality for the intricate electronic tasks it will perform. As the industry progresses, innovations in recycling and alternative materials sourcing are becoming increasingly important, reflecting a growing awareness of sustainability in semiconductor manufacturing.
Semiconductors serve as the malleable ‘clay’ that shapes the electronic components integral to our devices. Through doping—introducing specific impurities—these materials gain the ability to conduct electricity in precise ways, a cornerstone in the fabrication of diverse electronic components. This meticulous process underscores the semiconductor’s role as the brain of modern electronics, driving innovation across the technological landscape.
From Planar to FinFET: Evolution of Transistor Design
The transition from planar to FinFET transistor designs was a pivotal moment in semiconductor technology. FinFET, short for Fin Field-Effect Transistor, represents a major leap forward in addressing the challenges of scaling down devices while enhancing performance. This design significantly reduces leakage current, a critical issue as transistors become tinier and more densely packed.
Microchips utilizing FinFET gates first became commercialized in the early 2010s. They quickly became the dominant gate design at advanced nodes such as 14 nm, 10 nm, and 7 nm. The table below outlines the progression of FinFET adoption in commercial microchips:
Node (nm) | Adoption Year |
---|---|
14 | Early 2010s |
10 | Mid 2010s |
7 | Late 2010s |
These advancements were supported by the introduction of new materials like high-k dielectrics and metal gates, which further enhanced chip performance. As the industry continues to push the boundaries of Moore’s Law, FinFET technology remains at the forefront of innovation, setting the stage for the next generation of microelectronics.
Nanotechnology and the Future of Microelectronics
As the microelectronics industry approaches the limits of Moore’s Law, nanotechnology emerges as a beacon of innovation, offering new pathways to overcome existing barriers. The integration of nanoscience and nanoengineering is not just a trend; it’s a transformative shift that will define the future of microchips.
The potential applications of nanotechnology in microelectronics are vast and varied. Here are a few key areas where nanotechnology is making an impact:
- Enhanced performance: By reducing the size of transistors to the nanoscale, chips can operate faster and more efficiently.
- Increased capacity: Nanotechnology allows for a higher density of components, leading to more powerful and compact devices.
- Improved energy efficiency: Smaller components consume less power, which is crucial for portable and wearable technology.
- Advanced materials: The exploration of new semiconductor materials at the nanoscale could lead to breakthroughs in speed and functionality.
The journey towards integrating nanotechnology into microelectronics is filled with challenges, but the rewards promise to revolutionize the industry. As we stand on the brink of this new era, it is clear that the future trends in microelectronics will be heavily influenced by the advances in nanotechnology.
The Cutting Edge: Microchips in the 2020s
The Role of AI and Machine Learning in Chip Design
The intersection of artificial intelligence (AI) and machine learning with semiconductor technology marks a transformative era in chip design. Specialized chips, such as NVIDIA’s GPUs and Google’s TensorFlow Processing Units (TPUs), have emerged to meet the demands of processing vast datasets at unprecedented speeds. These chips are tailored for parallel processing, a necessity for the complex calculations required by machine learning algorithms.
Neuromorphic chips represent another innovative direction, drawing inspiration from the human brain to revolutionize AI applications. By emulating neural networks, these chips offer the potential for AI systems to learn and adapt dynamically, paving the way for more sophisticated and autonomous machine learning capabilities.
The role of AI extends beyond chip functionality, influencing the design process itself. AI-driven tools are now integral in optimizing chip architecture, leading to more efficient and powerful microelectronics. The synergy between AI and chip design not only enhances current technologies but also fuels the exploration of new frontiers in microelectronics.
Advanced Manufacturing Techniques
The miracle of modern chip manufacturing hinges on the precision and sophistication of advanced techniques. Only three companies in the world are at the forefront of this technological marvel, capable of producing the microchips that power today’s most advanced mobile devices and computing systems. These industry leaders, Intel, Samsung, and TSMC, have mastered the art of extreme ultraviolet (EUV) lithography, a process that has revolutionized the miniaturization of chips.
The process of creating microchips is intricate and multi-layered, involving several key steps:
- Designing Integrated Circuits (ICs)
- Analog design
- Digital design
- Mixed-signal design
- Fabricating ICs
- Making a base wafer
- Building layers through deposition and etching
- Photolithography
- Implantation
- Packaging the final product
Each step is critical, requiring exacting standards and precision engineering. The advent of EUV lithography has allowed for even finer patterning on chips, pushing the boundaries of what’s possible in microelectronics and leading to more powerful and efficient devices.
The Quest for Quantum Computing and Beyond
The pursuit of quantum computing marks a pivotal shift in the landscape of computational technology. Quantum computers leverage the peculiar properties of quantum bits, or qubits, to perform calculations at an unprecedented pace. This leap in processing power holds the promise of transformative advancements across various sectors, including cryptography, materials science, and drug discovery.
The journey towards realizing practical quantum computing has been marked by significant milestones. Companies like IBM, Google, and numerous startups are at the forefront of this technological race, striving to overcome the formidable challenges of scalability and error correction. The table below outlines a brief timeline of quantum computing‘s evolution, reflecting the rapid progress in this field.
Decade | Milestone |
---|---|
2010s | Crossroads of new computing paradigms |
2020s | Growing interest and investment in quantum computing |
As we confront the limitations imposed by Moore’s Law and the physical constraints of transistor miniaturization, the industry is compelled to explore alternative computing models. Quantum computing, along with other emerging technologies like neuromorphic computing, represents a paradigm shift that could redefine the capabilities of microchips and expand the horizons of what is computationally achievable.
Understanding Semiconductors: Principles and Applications
Doping Silicon and the P-N Junction
The art of semiconductor design begins with the doping of silicon, a process that introduces impurities into the pure silicon to modify its electrical properties. This deliberate contamination is essential for creating the p-n junction, the fundamental building block of most semiconductor devices.
Doping is carried out by adding specific elements to different areas of the silicon wafer, each chosen for its ability to donate or accept electrons. The result is the formation of p-type and n-type regions, which when combined, create the p-n junction. This junction is pivotal as it allows for the control of electrical current within a circuit.
The applications of the p-n junction are vast, ranging from simple diodes to complex integrated circuits. Below is a list of some key applications:
- Rectification in power supplies
- Signal processing in electronics
- Light emission in LEDs
- Solar energy conversion in photovoltaic cells
The Versatility of Field-Effect Transistors
Field-Effect Transistors (FETs) are pivotal in the realm of microelectronics, serving as the backbone of integrated circuits. Their ability to control the flow of current with high precision makes them indispensable in a myriad of applications. With their high input impedance, FETs ensure that the circuit’s performance remains consistent and reliable.
FETs come in various types, each suited for different applications. For instance, enhancement-mode FETs are widely used in digital circuits for their ability to switch states rapidly. On the other hand, Complementary metal-oxide semiconductors (CMOS) technology utilizes both n-type and p-type FETs to create low-power and high-efficiency circuits that are the cornerstone of modern computing devices.
The following table summarizes the key characteristics of different FET types:
FET Type | Switching Speed | Power Consumption | Application |
---|---|---|---|
Enhancement-mode | Fast | Low | Digital Circuits |
CMOS | Moderate | Very Low | Computing Devices |
As the demand for more powerful and energy-efficient devices grows, the role of FETs continues to expand. Their versatility allows for the integration of billions of transistors on a single chip, enabling complex computations and operations that power our modern devices.
Integrated Circuits: Complexity on a Tiny Scale
Integrated circuits (ICs), or microchips, are the cornerstone of modern electronics, encapsulating a multitude of electronic components into a minuscule space. Their ability to house billions of transistors has been pivotal in advancing technology, enabling the miniaturization of devices while boosting their capabilities.
Microchips, often no larger than a grain of rice, are the brains of nearly all electronic devices today. They perform complex calculations, process information, and manage the operations of other components within the systems they inhabit. This has been instrumental in the evolution of a wide array of technologies, from consumer electronics to sophisticated medical devices.
The production of ICs involves intricate processes and precision engineering. Below is a simplified overview of the key stages in the manufacturing of an integrated circuit:
- Design and simulation of the circuit
- Photolithography to imprint patterns on the silicon wafer
- Etching to remove excess material
- Doping to create p-n junctions
- Deposition of insulating, conducting, and semiconducting layers
- Packaging the chip for protection and integration
As we continue to push the boundaries of what’s possible with ICs, the complexity on this tiny scale only grows, leading to more powerful and efficient devices that continue to reshape our world.
Conclusion
The journey through the evolution of semiconductors has been a testament to human creativity and technological progress. From the early days of microelectronics to the cutting-edge microchips of the 2020s, each era has brought forth groundbreaking advancements that have revolutionized the way we live and work. The relentless pursuit of miniaturization, efficiency, and performance has driven the industry forward, with each breakthrough laying the foundation for the next. As we look to the future, the challenges of sustaining Moore’s Law and integrating new materials and nanotechnologies beckon a new wave of innovation. The semiconductor’s story is far from over; it is an ongoing saga of overcoming limitations and expanding the horizons of what is possible in the electronic world.
Frequently Asked Questions
What’s the difference between a semiconductor, a microchip, and an integrated circuit?
Semiconductors are materials with electrical conductivity between conductors and insulators, forming the foundation of electronic devices. Microchips, also known as microprocessors, are the ‘brains’ of modern electronics, containing millions of electronic components on a tiny silicon chip. Integrated circuits are complex assemblies of electronic components, including transistors, resistors, and capacitors, on a single chip, enabling the functionality of modern devices.
What is Moore’s Law?
Moore’s Law is the observation made by Gordon Moore in 1965 that the number of transistors on a microchip doubles approximately every two years, leading to a corresponding increase in computing power. This principle has driven the rapid advancement of electronic devices, although it faces challenges as physical limitations of transistor miniaturization are approached.
How are microchips, semiconductors, and integrated circuits made?
The creation of these components involves several steps: starting with the production of semiconductor materials, usually silicon, then shaping them through processes like photolithography and etching. Doping introduces impurities to modify electrical properties, and additional layers are added. Finally, the components are assembled, packaged, and undergo testing and quality control.
How has the introduction of new semiconductor materials impacted the industry?
The introduction of new semiconductor materials, such as high-k dielectrics and metal gates, has significantly improved chip performance by reducing leakage current and power consumption. These advancements have enabled the continuation of Moore’s Law and have been essential in meeting the demands of modern computing and electronic devices.
What role does nanotechnology play in the future of microelectronics?
Nanotechnology is critical in the development of smaller, faster, and more energy-efficient electronic components. By manipulating materials at the atomic and molecular level, nanotechnology allows for the creation of new semiconductor materials and transistor designs that push the boundaries of what’s possible in microelectronics.
What advancements can we expect in microchips in the 2020s?
The 2020s are expected to see continued advancements in AI and machine learning for chip design, more sophisticated manufacturing techniques like extreme ultraviolet lithography, and strides towards quantum computing. These developments will lead to more powerful, efficient, and specialized microchips that will drive innovation across various industries.