A Comprehensive Guide to Different Types of Semiconductors in Electronics
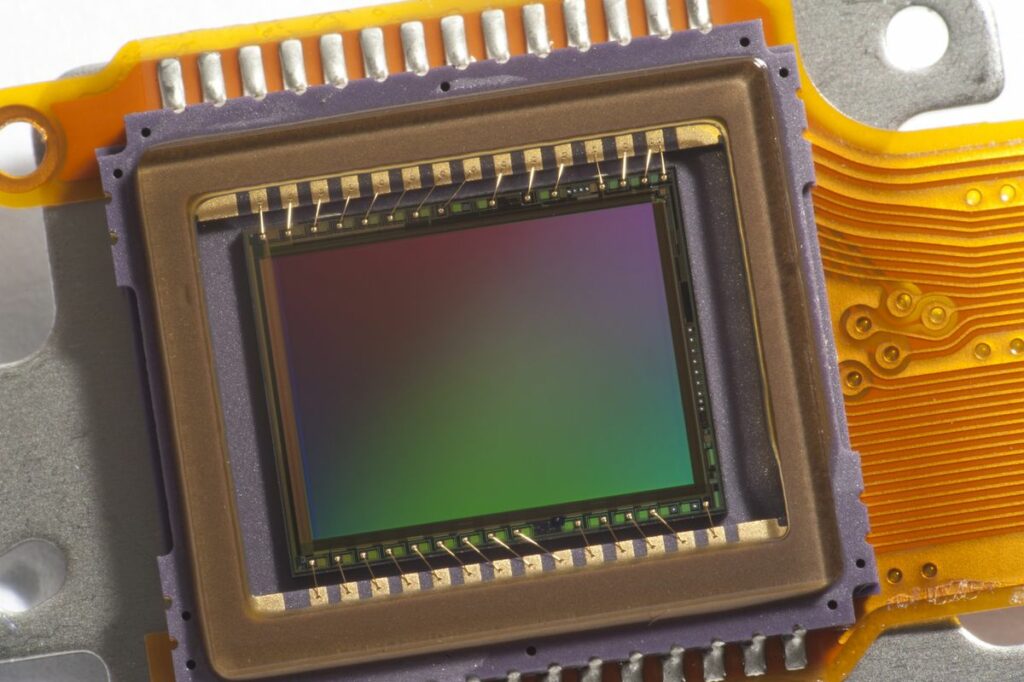
Semiconductors are the cornerstone of modern electronics, integral to devices we use every day. Understanding semiconductors is crucial for anyone interested in the field of electronics, as they are not just components but the very foundation upon which electronic systems are built. This guide explores the engineering principles, materials, devices, and applications of semiconductors, providing a thorough insight into their role in shaping the technology landscape.
Key Takeaways
- Semiconductors are essential for modern electronics, bridging the gap between conductors and insulators and enabling the function of devices from smartphones to satellites.
- Silicon remains the most widely used semiconductor material, but advancements in nanotechnology and compound semiconductors are enhancing device performance.
- Key semiconductor devices include diodes, transistors, and integrated circuits, with power semiconductors playing a crucial role in energy efficiency.
- Emerging technologies like Silicon Carbide (SiC) and Gallium Nitride (GaN) devices are pushing the boundaries of semiconductor performance, particularly in power applications.
- The semiconductor industry faces challenges such as supply chain issues and environmental impact, but is responding with innovations in manufacturing and sustainable practices.
Understanding Semiconductor Fundamentals
Definition and Importance of Semiconductors
Semiconductors are the cornerstone of modern electronics, integral to the function of a vast array of devices. They bridge the gap between conductors and insulators, offering a unique ability to control electrical conductivity under different conditions. This control is essential for the operation of semiconductor devices, which are electronic components that rely on the electronic properties of semiconductor materials.
The importance of semiconductors extends beyond their electrical properties. They are pivotal in advancing technology, from the simplest of gadgets to complex systems like satellites and smart cities. The versatility of semiconductors allows for their use in various applications, making them indispensable in the fields of computing, communication, and energy management.
Here are some key points highlighting the significance of semiconductors in today’s technological landscape:
- Semiconductors are fundamental to the creation of transistors, the basic building blocks of all modern electronic circuits.
- They enable the miniaturization of electronic components, leading to the development of compact, portable devices.
- Power semiconductors play a crucial role in energy efficiency, reducing power loss in devices ranging from personal computers to large-scale industrial equipment.
Conductivity and Band Theory
The behavior of semiconductors is governed by their ability to conduct electricity, which is explained through band theory. Band theory describes the energy levels of electrons in a material and is crucial for understanding how semiconductors function. In semiconductors, the energy gap between the valence band (full of electrons) and the conduction band (where electrons can move freely) is small enough that electrons can jump the gap when energy is applied.
This energy gap, known as the band gap, determines a material’s electrical conductivity. A smaller band gap means that less energy is required for an electron to move into the conduction band, making the material more conductive. Conversely, a larger band gap requires more energy, making the material less conductive. The following list outlines the effects of temperature and doping on the conductivity of semiconductors:
- Temperature: As temperature increases, more electrons gain the energy needed to cross the band gap, leading to increased conductivity.
- Doping: Introducing impurities, or ‘doping’, can add free carriers in the form of electrons or holes, thus altering the material’s conductivity.
Understanding these principles is essential for designing and optimizing semiconductor devices for various electronic applications.
Intrinsic and Extrinsic Semiconductors
Semiconductors are categorized into two main types: intrinsic and extrinsic. Intrinsic semiconductors are pure forms of semiconductor materials without any significant impurity atoms. They exhibit a balanced number of electrons and holes and have a conductivity that is highly temperature-dependent. On the other hand, extrinsic semiconductors are doped with impurities to alter their electrical properties.
The process of doping introduces additional free charge carriers, either electrons (n-type) or holes (p-type), which significantly increases the material’s conductivity. The choice between intrinsic and extrinsic semiconductors is determined by the intended application. For instance, photovoltaic cells (PVCs), which convert sunlight into electricity, typically use extrinsic semiconductors to enhance efficiency.
Here’s a simple comparison:
- Intrinsic: Pure, undoped, balanced charge carriers, temperature-sensitive conductivity
- Extrinsic: Doped, additional charge carriers, enhanced conductivity
Materials and Fabrication of Semiconductors
Silicon, Germanium, and Compound Semiconductors
Silicon and germanium are the foundational materials in the semiconductor industry, with silicon being the most widely used due to its abundance and favorable electrical properties. Germanium was the first material to be used in semiconductors, but it has largely been replaced by silicon because of its temperature sensitivity and higher cost.
Compound semiconductors, on the other hand, are composed of two or more elements. They often exhibit superior properties for specific applications, such as higher electron mobility or direct bandgap. These materials include gallium arsenide (GaAs), indium phosphide (InP), and silicon carbide (SiC), among others. The choice of semiconductor material affects the performance, efficiency, and application of the final electronic device.
Here is a comparison of key properties of these semiconductor materials:
Material | Bandgap (eV) | Electron Mobility (cm^2/Vs) | Thermal Conductivity (W/mK) |
---|---|---|---|
Silicon (Si) | 1.12 | 1500 | 150 |
Germanium (Ge) | 0.66 | 3900 | 60 |
Gallium Arsenide (GaAs) | 1.43 | 8500 | 55 |
Silicon Carbide (SiC) | 3.26 | 900 | 490 |
The development of nanotechnology in semiconductor manufacturing has enabled the creation of materials with tailored properties, pushing the boundaries of what’s possible in electronics. This advancement is crucial for the miniaturization and performance enhancement of semiconductor devices.
Nanotechnology in Semiconductor Manufacturing
The advent of nanotechnology has revolutionized semiconductor manufacturing, enabling the creation of materials and devices at the atomic and molecular scale. This precision allows for the development of semiconductors with superior electrical properties and higher performance.
Nanotechnology’s role in semiconductor fabrication involves several key techniques:
- Top-down nanofabrication: Traditional lithography and etching methods are used to create nanostructures.
- Bottom-up nanofabrication: Chemical processes assemble devices atom by atom or molecule by molecule.
- Nanoscale characterization: Tools like scanning electron microscopes (SEMs) and atomic force microscopes (AFMs) are used to analyze materials at the nanoscale.
These methods contribute to the production of smaller, faster, and more energy-efficient semiconductor devices. As the industry strives for further miniaturization, nanotechnology remains at the forefront, pushing the boundaries of what’s possible in electronic components.
Doping Processes and Material Properties
The doping process is a critical step in semiconductor fabrication, where impurities are intentionally introduced to alter the electrical properties of the base material. This process allows for the precise control of the semiconductor’s conductivity, enabling the creation of p-type or n-type materials, which are essential for the function of various electronic devices.
Material properties play a significant role in the performance of semiconductors, especially in high-frequency applications such as RF PCBs (Radio Frequency Printed Circuit Boards). Materials with low dielectric constants and loss tangents are preferred to minimize signal loss and support higher frequency operations. The choice of substrate material, such as the emerging liquid crystal polymer (LCP), can greatly influence the thermal stability and electrical performance at extremely high frequencies.
When selecting materials for RF PCBs, designers must consider a balance between cost, performance, and manufacturability. The decision impacts not only the efficiency of signal transmission but also the overall functionality and reliability of the final product.
Semiconductor Devices and Applications
Diodes, Transistors, and Integrated Circuits
Diodes, transistors, and integrated circuits (ICs) are the fundamental building blocks of modern electronics. Diodes allow current to flow in one direction, serving as essential components in power conversion and signal processing. Transistors, on the other hand, act as switches or amplifiers and are the backbone of digital logic in computing and communication systems.
Integrated circuits combine multiple semiconductor devices into a single package, drastically reducing size and power consumption while increasing reliability and functionality. The evolution from single transistors to complex ICs has enabled the miniaturization of electronic devices, leading to the proliferation of portable and wearable technology.
Here is a list of common semiconductor devices and their typical applications:
- MOSFETs: Power regulation and switching
- Diodes: Rectification and signal modulation
- Bipolar Transistors: Amplification and switching
- Microcontrollers: Central processing in embedded systems
- Sensors: Environmental monitoring and user interaction
Each device type plays a distinct role in electronic circuits, and ongoing advancements continue to expand their capabilities and applications.
Power Semiconductors and Energy Efficiency
Power semiconductors are pivotal in managing and converting electrical power efficiently, which is essential for minimizing energy loss in a myriad of electronic devices. From personal computers to smartphones, and from automotive applications to electrical substations, the role of power semiconductors is to ensure that power loss is kept to a minimum, thereby saving energy.
The advancement in power semiconductor technology, particularly with the introduction of Silicon Carbide (SiC) devices, has led to significant improvements in energy efficiency. SiC MOSFETs, for example, are known for their high dielectric breakdown strength, which is about 10 times higher than that of silicon. This allows for high withstand voltages and low on-resistance, enabling devices to achieve high switching speeds while reducing power consumption.
Recent developments in the industry have seen the expansion of SiC MOSFET modules, which contribute to the high efficiency and downsizing of industrial equipment. These modules are becoming increasingly important in the design of power supply systems, including 3-phase AC converters and bidirectional DC-DC converters, which are critical for the operation of high-performance electronic systems.
Feature | Benefit |
---|---|
High dielectric breakdown strength | Allows high withstand voltages |
Low on-resistance | Reduces power consumption |
High switching speeds | Improves efficiency of power systems |
The future of energy-efficient electronics relies heavily on the continuous innovation and implementation of advanced power semiconductors, which are indeed the brain of energy efficiency in the digital economy.
Application-Specific Integrated Circuits (ASICs)
Application-Specific Integrated Circuits (ASICs) represent a pinnacle in customization for electronic devices. They are designed to perform a specific function and are optimized for that purpose, which can lead to significant improvements in performance and power efficiency compared to general-purpose processors.
ASICs are prevalent in a variety of industries, from consumer electronics to complex data centers. For instance, in smartphones, ASICs enable a multitude of features such as image processing and battery management, all while maintaining a compact form factor. In the realm of cryptocurrency, ASICs are tailored for mining, providing unmatched efficiency.
The following list outlines some common applications of ASICs:
- Smartphones and wearable devices
- High-performance servers
- Cryptocurrency mining hardware
- Automotive control systems
- Medical devices
As technology advances, the role of ASICs continues to expand, offering tailored solutions that address the specific needs of emerging applications.
Advancements in Semiconductor Technology
Silicon Carbide (SiC) and Gallium Nitride (GaN) Devices
Silicon Carbide (SiC) and Gallium Nitride (GaN) are at the forefront of semiconductor technology, known for their wide-bandgap properties that achieve high switching speeds and reduced power consumption. These materials are revolutionizing power device advancements, offering significant benefits over traditional silicon-based devices.
SiC devices, such as Metal-Oxide-Semiconductor Field-Effect Transistors (MOSFETs) and Schottky Barrier Diodes (SBDs), are renowned for their high dielectric breakdown strength, which is approximately 10 times greater than that of silicon. This allows for devices with high withstand voltages and low on-resistance, making them suitable for power supply circuits and high-power applications like inverters and converters.
GaN devices also exhibit exceptional performance in terms of efficiency and thermal management, making them ideal for applications in the automotive and aerospace industries. The table below compares some key features of SiC and GaN devices:
Feature | SiC Devices | GaN Devices |
---|---|---|
Breakdown Strength | High (10x Si) | Moderate |
On-Resistance | Low | Very Low |
Switching Speed | Fast | Very Fast |
Thermal Conductivity | Good | Excellent |
Application Examples | Power supply circuits, Inverters | Automotive, Aerospace |
The advancements in these wide-bandgap semiconductors are not only enhancing the capabilities of electronic devices but also contributing to energy efficiency and sustainability in various sectors.
The Role of Semiconductors in IoT and Robotics
Semiconductors are the cornerstone of modern IoT and robotics, enabling the integration of advanced technologies such as AI and machine learning. These components facilitate the development of smarter, more efficient, and highly autonomous systems. The synergy between semiconductors and IoT leads to enhanced data processing capabilities and connectivity, which are essential for the real-time exchange of information and remote monitoring.
Key advancements in semiconductor technology that impact IoT and robotics include:
- Integration of AI and ML: Semiconductors enable robots to learn and adapt, enhancing efficiency and versatility.
- Collaboration between humans and robots: Semiconductors are at the heart of systems that allow for safe and productive human-robot collaboration.
- Advancements in vision systems and sensors: These improvements are crucial for precise object detection and manipulation, opening new avenues in various industries.
As industries embrace digital transformation, companies like ABB Robotics are leveraging semiconductors to shape the future of industrial automation. The integration of semiconductors into the Industrial Internet of Things (IIoT) ecosystem is pivotal for optimized operations and improved decision-making, particularly in motion control and robotics.
Future Trends: 3D Printing and Flexible Electronics
The realm of electronics is on the cusp of a transformative era with the advent of 3D printing and flexible electronics. These technologies herald a future where electronics are not only ubiquitous but also conform to the dynamic needs of various industries.
Flexible electronics, particularly in the form of Radio Frequency Printed Circuit Boards (RF PCBs), are revolutionizing wearable devices. Materials like polyimide and liquid crystal polymer (LCP) are key to this innovation, providing the necessary electrical properties and the ability to flex without damage. The applications are vast, from smart clothing to medical monitors, all benefiting from the seamless integration with daily life.
Additive manufacturing, including 3D printing of RF circuits, is another groundbreaking trend. It enables the creation of complex structures and the efficient production of customized RF PCBs. This method is not only cost-effective but also enhances design flexibility, allowing for small batch production without the traditional manufacturing constraints.
As these technologies mature, we can anticipate a surge in novel applications that blend electronics with everyday objects, making the future of electronics both exciting and accessible.
Challenges and Solutions in Semiconductor Industry
Supply Chain and Long-Term Availability
The semiconductor supply chain is a complex and multifaceted system that is crucial for the production and distribution of electronic devices. Recent updates have highlighted the influence of geopolitics, national security, and government incentives on the market. These factors can lead to disruptions, but also present opportunities for strategic adjustments and resilience building.
To ensure long-term availability, companies are exploring various strategies. Some of these include:
- Diversifying sources of raw materials and manufacturing locations
- Investing in supply chain analytics and AI-based technologies for better forecasting and risk management
- Establishing partnerships and alliances to secure critical components
- Advocating for supportive policies and incentives from governments
The literature underscores the importance of resilience in the semiconductor supply chain. Studies by Bechtsis et al. (2021) and Belhadi et al. (2021) suggest that data-driven and AI-enhanced techniques can significantly bolster supply chain robustness. Furthermore, frameworks for strategic data management, as proposed by Bonvino and Giorgino (2024), can create competitive value and mitigate vulnerabilities.
Environmental Impact and Sustainability Efforts
The semiconductor industry faces significant environmental challenges, particularly in terms of resource consumption and waste management. Sustainable practices are becoming increasingly crucial as the industry seeks to minimize its ecological footprint. Recent studies highlight the role of big data analytics and artificial intelligence in enhancing green supply chain processes and improving environmental performance.
Efforts to reduce environmental impact include the adoption of circular economy principles, where materials are reused and recycled, thus extending the lifecycle of semiconductor products. Predictive maintenance strategies, powered by machine learning, contribute to this by optimizing the use of resources and reducing waste.
Year | Study | Key Focus |
---|---|---|
2022 | S. Bag et al. | Sustainable manufacturing practices and circular economy capabilities |
2021 | S. Benzidia et al. | Green supply chain process integration |
2022 | M.A. Algarni et al. | Linking environmental behavior with financial performance through corporate sustainability |
These initiatives not only support environmental sustainability but also have the potential to improve financial performance, creating a compelling case for businesses to invest in eco-friendly technologies and processes.
Innovation in Semiconductor Packaging and Assembly Techniques
The relentless pursuit of miniaturization and performance enhancement in the semiconductor industry has led to significant innovations in packaging and assembly techniques. Advanced soldering techniques, such as reflow soldering, have become crucial in addressing the challenges posed by the assembly of sensitive components like RF modules. These methods ensure a reliable connection while minimizing thermal stress, which is vital for maintaining signal integrity and component longevity.
The evolution of packaging technology is also evident in the automotive sector, where the integration of small-geometry trench process technology with low-resistance packaging has resulted in MOSFETs with industry-leading low on-resistance. Innovations such as the use of a Cu connector bonding technique have doubled the current-carrying capacity compared to conventional models, showcasing the importance of packaging in enhancing device performance.
As semiconductor packaging contributes to cost efficiency, it’s essential to recognize its role in simplified manufacturing processes, reduced material costs, and increased production yield. The table below summarizes the impact of innovative packaging techniques on key performance indicators:
Technique | Performance Improvement | Cost Reduction |
---|---|---|
Reflow Soldering | Enhanced Signal Integrity | Lower Thermal Stress |
Cu Connector Bonding | Increased Current Capacity | Reduced On-Resistance |
Conclusion
In this comprehensive guide, we have explored the multifaceted world of semiconductors, which are the cornerstone of modern electronics. From the basic engineering principles to the advanced applications in devices such as smartphones, satellites, and power systems, semiconductors play a pivotal role in the technological advancements of our time. We delved into various semiconductor materials, their properties, and how they are harnessed in different types of devices like transistors, diodes, and ASICs. Understanding these components is crucial for anyone looking to grasp the intricacies of electronics and their impact on our daily lives. As we continue to push the boundaries of what’s possible with nanotechnology and power semiconductors, the future of electronics looks bright, promising even more sophisticated and energy-efficient solutions.
Frequently Asked Questions
What is a semiconductor?
A semiconductor is a material with electrical conductivity between that of a conductor and an insulator. It is the foundation of modern electronics, used in devices like smartphones, satellites, and computers to control the flow of electricity.
How does doping affect a semiconductor?
Doping introduces impurities into a semiconductor to change its electrical properties. N-type doping adds electrons, while P-type doping creates holes. This process allows for the control of electrical conductivity and the creation of semiconductor devices like diodes and transistors.
What are the main materials used in semiconductor fabrication?
Silicon and germanium are the primary materials used in semiconductor fabrication. Compound semiconductors, which are made from two or more elements, are also important, especially in high-performance applications.
What role do power semiconductors play in electronics?
Power semiconductors control and convert electrical power efficiently, reducing power loss in devices such as personal computers, smartphones, and automotive applications. They are crucial for energy-saving technologies.
What is an ASIC and why is it important?
An Application-Specific Integrated Circuit (ASIC) is a type of semiconductor device designed for a specific use or application. ASICs are important because they can be optimized for performance, power efficiency, and size, making them ideal for dedicated functions in electronics.
What are the future trends in semiconductor technology?
Future trends in semiconductor technology include the development of Silicon Carbide (SiC) and Gallium Nitride (GaN) devices, flexible electronics, and the integration of semiconductors with 3D printing technologies. These advancements promise to enhance performance and enable new applications.