Demystifying Semiconductor Equipment: Tools of the Trade for Modern Electronics
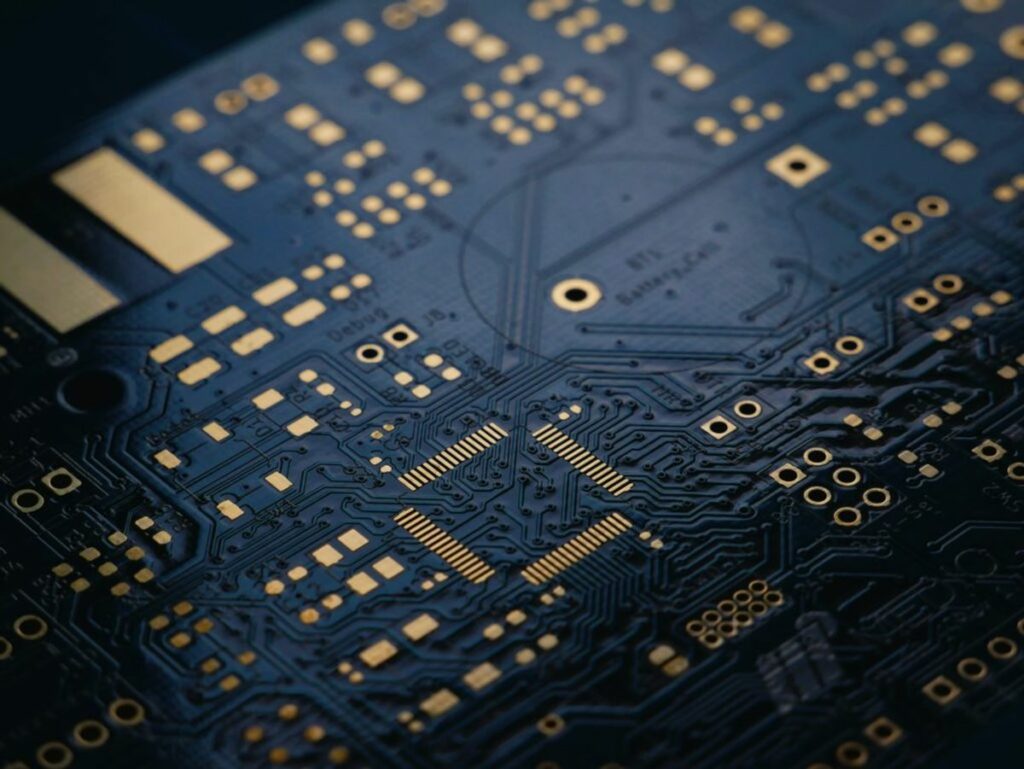
The realm of semiconductor equipment is intricate and essential for the advancement of modern electronics. This article, ‘Demystifying Semiconductor Equipment: Tools of the Trade for Modern Electronics,’ delves into the tools and strategies that are at the forefront of semiconductor technology. From optimizing switch performance to mitigating electromagnetic interference (EMI), we explore the innovations shaping the industry. We also examine the impact of new semiconductor materials and design techniques that are essential for ensuring device safety and performance in the ever-evolving landscape of connectivity and the Internet of Things (IoT).
Key Takeaways
- Fast edge rates in semiconductor switches can lead to EMI issues, which can be mitigated with good design practices and the use of snubbers.
- Tools like the FET-Jet Calculator V2 aid in the selection and visualization of SiC FET and diode performance for efficient power design.
- Advancements in materials such as GaN and SiC are revolutionizing power electronics and RF applications, balancing cost and performance.
- Designing for electromagnetic compatibility (EMC) is crucial, involving strategies like ESD design for mobile devices and minimizing switching loss in SiC FETs.
- Semiconductor equipment plays a vital role in connectivity and IoT, with tools and components such as attenuators, phase shifters, and frequency converters enabling versatile communication.
Understanding Semiconductor Switches and Minimizing EMI
The Ideal Semiconductor Switch: Balancing Performance and EMI
In the pursuit of the ideal semiconductor switch, designers face the challenge of balancing fast switching speeds with the minimization of electromagnetic interference (EMI). Fast edge rates are crucial for reducing switching losses and achieving higher efficiency, but they can exacerbate EMI concerns. These issues often manifest as unwanted oscillations and voltage spikes, which can be mitigated with careful design and the use of snubbers.
Another aspect to consider is the trade-off between performance and design complexity. As switches approach ideal behavior with hyper-fast edge rates, the potential for voltage overshoots and ringing increases. This necessitates the implementation of solutions such as simple RC snubbers, particularly for Silicon Carbide (SiC) FETs, to maintain efficiency gains while addressing these challenges.
The table below outlines key considerations when selecting a semiconductor switch for applications such as electric vehicle power converters:
Factor | Consideration |
---|---|
Parallel Device Number and Rating | Optimize based on power requirements |
Switching Frequency | Balance with efficiency targets |
Operating Mode | Choose based on application needs |
Efficiency Target | Set to meet system goals |
Junction Temperature Rise | Keep within acceptable limits |
Loss Apportioning | Distribute between conduction and switching |
Cost | Align with budget constraints |
Techniques like Spread Spectrum Frequency Modulation and Silent Switcher™ technology are also employed to reduce EMI effectively. For instance, the LT8640 is a unique component that leverages layout design to minimize EMI, demonstrating the innovative approaches in modern semiconductor equipment.
Snubbers: Tackling Oscillations and Voltage Spikes
In the quest for the ideal semiconductor switch, engineers face the challenge of balancing performance with the suppression of unwanted electrical phenomena. Fast edge rates, while beneficial for reducing switching losses and improving power density, can exacerbate issues with electromagnetic interference (EMI). These issues often manifest as oscillations and voltage spikes, which can be detrimental to sensitive electronic components.
Snubber circuits are the unsung heroes in this scenario. They are specifically designed to suppress these voltage spikes that occur due to sudden changes in current flow. A well-designed snubber can be a small yet critical component in a power design, ensuring stability and protecting against potential damage.
Here’s a brief overview of the types of snubbers commonly used in semiconductor applications:
- RC (Resistor-Capacitor) Snubbers: Used for damping and controlling the rate of voltage change.
- RCD (Resistor-Capacitor-Diode) Snubbers: Provide better energy efficiency by allowing energy recirculation.
- LC (Inductor-Capacitor) Snubbers: Effective for high-power applications, offering superior energy storage.
Understanding the working principle and applications of snubber circuits is essential for any engineer looking to optimize semiconductor switch performance while minimizing EMI-related issues.
Design Strategies to Mitigate EMI in High-Frequency Circuits
In the realm of high-frequency circuit design, mitigating electromagnetic interference (EMI) is a critical challenge that engineers face. The rapid edge rates in modern semiconductor switches, while beneficial for reducing switching losses and improving power density, can exacerbate EMI concerns. These issues often manifest as unwanted oscillations and voltage spikes, which can be mitigated through careful PCB layout and the use of snubbers.
Effective EMI and EMC design strategies are essential for ensuring device compatibility and reducing interference. A structured approach to PCB layout can significantly diminish the risk of EMI-related problems. Below is a list of strategies that can be employed to achieve this:
- Utilize snubbers to control voltage spikes and oscillations.
- Implement careful routing of traces to minimize parasitic inductances.
- Employ shielding and proper grounding techniques to prevent noise propagation.
- Opt for components with favorable EMI characteristics.
By adhering to these strategies, designers can enhance the performance of high-frequency circuits while maintaining compliance with EMI and EMC standards.
Tools for Optimizing Semiconductor Selection and Performance
FET-Jet Calculator: Streamlining SiC FET and Diode Selection
The FET-Jet Calculator V2 has emerged as an indispensable tool for engineers looking to optimize their power design with SiC FETs and Schottky diodes. By providing a user-friendly interface, the calculator simplifies the selection process and offers a visual representation of device performance. This ensures that designers can make informed decisions quickly, leading to more efficient first-pass designs.
UnitedSiC’s FET-Jet Calculator eliminates the guesswork in SiC FET selection, making it easier for designers to find the power transistors that best fit their design goals. It not only aids in device selection but also predicts system performance with a high degree of accuracy. This is crucial in a field where efficiency and cost are tightly interwoven, and the right choice can significantly enhance the performance of power converters.
The advantages of using the FET-Jet Calculator include:
- Simplified device selection process
- Accurate predictions of system performance
- Visualization of device performance characteristics
- Assistance in achieving a balance between fast switching speeds and design complexity
As the power electronics market continues to evolve, tools like the FET-Jet Calculator are vital for staying ahead of the curve and unleashing the full potential of SiC technology.
Achieving Wi-Fi Tri-Band Gigabit Speeds: Component Challenges
The quest for Wi-Fi tri-band gigabit speeds is a complex endeavor, involving a myriad of component challenges. Engineers must navigate the intricate balance between performance, thermal management, and the coexistence of multiple frequency bands.
One of the pivotal aspects is the thermal challenges in Wi-Fi front-end designs. As devices strive for higher speeds and performance, they generate more heat, necessitating innovative cooling solutions to maintain optimal operation.
Moreover, the introduction of Wi-Fi 6E/7 adds an extra 1200 MHz of bandwidth, which brings about the need for Automated Frequency Coordination (AFC). This system ensures harmonious coexistence with other services in the expanded spectrum, such as licensed operational fixed, common carrier, and television services.
To illustrate the complexity, here’s a list of key component challenges:
- Balancing high performance with thermal constraints
- Ensuring reliable operation across all frequency bands
- Implementing effective AFC to manage the expanded spectrum
- Overcoming interference from other wireless services
Addressing these challenges is critical for achieving the high-speed connectivity that modern applications demand.
SEED Methodology: Enhancing ESD RF Front-End Design
The SEED (Simulation for Enhanced ESD Design) methodology is a powerful approach for optimizing the ESD (Electrostatic Discharge) protection in RF (Radio Frequency) front-end designs. By leveraging advanced modeling and simulation techniques, engineers can predict how a mobile device will respond to system-level IEC stress tests before the physical PCB (Printed Circuit Board) layout begins. This preemptive analysis is crucial for ensuring that the device meets stringent ESD protection standards and functions reliably in the field.
The SEED methodology encompasses a variety of tools and strategies. For instance, it guides the selection of on-board components for ESD protection and outlines the best practices for safeguarding mobile system designs. Here’s a brief overview of the key components involved in SEED:
- ESD protection devices
- RF switches
- Antenna matching networks
- Signal integrity analysis tools
By integrating these components effectively, designers can enhance the ESD robustness of their devices while maintaining optimal RF performance. The following table summarizes the device ESD design sensitivity trends based on prevalent ESD models used by manufacturers:
Year | HBM (Human Body Model) | CDM (Charged Device Model) |
---|---|---|
2018 | 2 kV | 500 V |
2019 | 1.5 kV | 250 V |
2020 | 1 kV | 100 V |
As the table indicates, there is a clear trend towards increased sensitivity to ESD events, necessitating more robust design strategies. The SEED methodology is instrumental in addressing these challenges and ensuring that RF front-end designs are not only compliant but also resilient in the face of ESD phenomena.
Advancements in Semiconductor Materials and Their Impact
GaN and SiC: Revolutionizing Power Electronics and RF Applications
The advent of wide band-gap semiconductors such as gallium nitride (GaN) and silicon carbide (SiC) has marked a significant turning point in the field of power electronics and RF applications. These materials are known for their exceptional power conversion efficiency and compact form factors, outshining traditional silicon-based components. Qorvo’s SiC-FET technology, for instance, showcases a 750V device in a remarkably small TO-Leadless (TOLL) package, offering substantial benefits for design optimization.
GaN, in particular, has emerged as the preferred choice for high-RF, power-intensive applications, thanks to its superior performance over silicon and gallium arsenide (GaAs). The integration of UnitedSiC by Qorvo has broadened the company’s influence in burgeoning markets such as electric vehicles (EVs), industrial power, and data centers. Below is a list highlighting the key advantages of GaN and SiC in modern applications:
- Higher power density and efficiency
- Faster switching speeds
- Enhanced thermal performance
- Smaller size for space-constrained designs
- Increased reliability in harsh environments
These advancements in semiconductor technology are not just theoretical; they are being actively implemented across various sectors, driving innovation and efficiency to new heights.
65V GaN Technology: Transforming the Radar Market
The introduction of 65 Volt GaN technology is a game-changer for radar applications, particularly in the L-Band and IFF (Identification Friend or Foe) systems. Its superior signal integrity and extended reach are pivotal for the next generation of radar systems, offering enhanced performance and capabilities.
GaN technology has been pivotal in the evolution of radar systems, providing higher power, wider bandwidth, and high breakdown voltage performance. These characteristics make GaN an ideal choice for phased array radar systems, which require precise power management and the ability to operate across multiple frequency bands.
The benefits of GaN extend beyond just technical specifications. The cost-benefit relationship of integrating GaN into radar systems is increasingly favorable, as continuous improvements make it a cost-competitive option compared to older semiconductor technologies. As the radar market continues to grow, the impact of 65V GaN technology cannot be overstated, with its ability to revolutionize radar design and contribute to the significant growth in the global radar industry.
Continuous Improvement in SiC Semiconductors: Cost vs. Performance
The evolution of Silicon Carbide (SiC) semiconductors is a testament to the relentless pursuit of balancing cost with performance. As power electronics demand more efficient and high-performing components, SiC FETs have emerged as a significant player. They offer a combination of high power levels, fast switching speeds, and superior thermal properties compared to traditional silicon-based parts.
The cost-benefit relationship in SiC technology is complex, yet the trajectory is clear: continuous improvements are making SiC devices more cost-competitive. This is crucial for power engineers who must navigate the ever-changing landscape of power electronics. The table below illustrates the performance gains and cost reductions over recent years:
Year | Efficiency Improvement | Cost Reduction |
---|---|---|
2020 | 5% | 3% |
2021 | 7% | 5% |
2022 | 10% | 7% |
2023 | 12% | 9% |
Innovations such as the SiC ‘cascode’ FET are pushing the boundaries further, offering better system performance and minimizing EMI and switching loss. The industry’s commitment to innovation ensures that SiC technology will continue to be a totemic symbol in the realm of power electronics.
Electromagnetic Compatibility: Designing for Device Safety
ESD Design Strategies for Mobile Devices
In the realm of mobile device design, Electrostatic Discharge (ESD) protection is paramount for ensuring the longevity and reliability of the device. ESD can cause immediate damage or degrade components over time, leading to device failure. As such, designers must employ strategic measures to safeguard against these potentially destructive discharges.
One effective approach is the implementation of on-board components specifically designed for ESD protection. These components act as a shield, absorbing and diverting the energy away from sensitive parts of the mobile system. Additionally, the SEED (System Efficient ESD Design) methodology offers a structured framework for optimizing ESD protection in the RF front-end design. By utilizing modeling and simulation, designers can predict the performance of ESD protection schemes and ensure they meet system-level IEC stress testing standards before the physical layout of the phone’s PCB is finalized.
Moreover, with the evolution of manufacturing capabilities and standards, there is now increased flexibility in ESD protection design for components such as smartphone antenna tuners. This allows for more creative and space-efficient solutions in the ever-compact mobile device form factors. Designers are encouraged to explore innovative techniques to integrate new features without compromising on the available space or the device’s ESD defense mechanisms.
System-Level IEC Stress Testing: Ensuring ESD Protection
Ensuring that mobile devices can withstand electrostatic discharges (ESDs) is critical for their longevity and reliability. System-level IEC stress testing is a rigorous process that evaluates a device’s resilience to ESD events. This testing simulates real-world scenarios where indirect contact-discharge can occur, providing valuable insights into the robustness of the device’s ESD protection mechanisms.
The IEC 61000-4 standard outlines the requirements for ESD immunity testing. It specifies the parameters for the test setup, including the energy and waveform of the discharge, as well as the points of application. A typical measurement setup for this test is detailed in Fig. 4, which illustrates the indirect contact-discharge test mode. By adhering to these standards, manufacturers can ensure that their devices are prepared to handle unexpected ESD events without failure.
To facilitate this testing, a structured approach is often employed, involving the following steps:
- Identification of ESD-sensitive components
- Selection of appropriate ESD protection devices
- Implementation of a test plan based on IEC standards
- Analysis of test results to identify potential vulnerabilities
- Iterative design improvements to enhance ESD resilience
By systematically addressing each of these areas, designers can optimize their products to pass system-level IEC stress tests, thereby increasing the confidence of consumers in the durability of their mobile devices.
Minimizing Switching Loss in SiC FETs for Enhanced EMC
The quest for efficiency in power electronics has led to the adoption of Silicon Carbide (SiC) Field-Effect Transistors (FETs), known for their ability to operate at higher voltages and currents. This characteristic not only increases power density but also significantly reduces switching losses, which is crucial for maintaining electromagnetic compatibility (EMC).
To further minimize switching losses in SiC FETs, designers are implementing various strategies. One such approach is the use of RC snubbers, which help to mitigate voltage overshoots and ringing effects that can occur with fast edge rates. Additionally, the Kelvin connection technique is employed to reduce inductance in the circuit, enhancing the switching efficiency of SiC FETs packaged in low thermal resistance housings like the TO-247.
Here’s a brief overview of the benefits of optimizing SiC FETs for reduced switching losses:
- Increased power conversion efficiency: By reducing losses, the overall efficiency of power conversion is improved.
- Enhanced EMC performance: Lower switching losses contribute to reduced EMI, which is vital for device safety and compliance with regulatory standards.
- Improved thermal management: Minimizing losses leads to less heat generation, allowing for better thermal management and potentially smaller heat sinks.
The Role of Semiconductor Equipment in Connectivity and IoT
Connectivity Q&A: Understanding IoT Network Standards
In the realm of IoT, understanding network standards is crucial for seamless communication between devices. The Internet of Things (IoT) is a complex ecosystem, involving a variety of technologies, protocols, and devices that must work in harmony. Tony Testa simplifies this complexity in his Q&A blog posts, providing key insights into IoT network standards.
IoT data protocols play a pivotal role in formatting data for communication across the IoT network. These protocols ensure that devices, regardless of their make or model, can exchange information effectively. The advancement of IoT devices is further propelled by the integration of mesh network topologies and ultra-wideband technology, expanding the possibilities for IoT applications.
ConcurrentConnect technology is a game-changer for multi-standard IoT communications, offering a unique solution to the challenges posed by the diverse mix of communication standards in home networking. Energy efficiency is another significant impact of IoT, with the potential to transform everything from commercial buildings to household appliances. As we continue to explore the IoT landscape, it’s clear that cellular networks are key to unlocking the full potential of IoT devices, enabling them to work together to enhance our daily lives.
RF Power Management: Attenuators, Limiters, and Phase Shifters
In the realm of RF power management, attenuators, limiters, and phase shifters play pivotal roles in ensuring signal integrity and controlling power levels within communication systems. Attenuators are used to reduce signal strength, limiters protect against signal overloads, and phase shifters adjust the phase of signals to achieve desired timing or to nullify interference.
For instance, the PE44820 – RF Digital Phase Shifter, a product by pSemi, exemplifies the sophistication of modern phase shifters. It operates within the 1.7–2.2 GHz frequency band and offers a phase range of 358.6° in 1.4° steps, ensuring excellent phase and amplitude accuracy.
The selection of these components is critical for the performance of various systems, from radar to communication networks. Below is a list of control products related to RF power management:
- Attenuators
- Limiters
- Phase Shifters
- Programmable Capacitor Arrays
Each component category serves a specific function and is integral to the overall system’s reliability and efficiency.
Frequency Converters and Sources: Enabling Versatile Communication
In the realm of modern electronics, frequency converters and sources play a pivotal role in enabling communication across various platforms and standards. These components are essential for translating signals into the appropriate frequency bands required for different communication protocols, ensuring interoperability and efficient data transmission.
Frequency converters, including upconverters and downconverters, are used to adjust the frequency of a signal to match the requirements of the communication system. Sources such as integrated synthesizers with mixers, modulators, and multipliers provide the necessary signal generation and manipulation to facilitate a wide range of applications.
The following table summarizes some key components and their functions in RF communication systems:
Component | Function |
---|---|
Mixers | Combine or separate signals by frequency |
Modulators | Impose data onto a carrier wave |
Multipliers | Increase frequency for higher-band applications |
Upconverters | Convert low-frequency signals to higher frequencies |
Downconverters | Convert high-frequency signals to lower frequencies |
Conclusion
In the journey through the intricate landscape of semiconductor equipment, we have uncovered the pivotal tools that empower modern electronics. From the precision of FET-Jet Calculators to the robustness of SiC FETs and GaN HEMTs, these components are the building blocks of today’s high-speed, energy-efficient devices. We’ve explored how designers navigate the challenges of EMI, switching losses, and ESD protection, employing innovative strategies and simulation tools to optimize performance. As the industry continues to evolve, driven by the relentless pursuit of miniaturization and cost-effectiveness, the role of advanced semiconductor equipment becomes ever more critical. Understanding these tools is not just about grasping the current state of technology but also about envisioning the future of electronics where innovation and practicality converge.
Frequently Asked Questions
What are the challenges of an ideal semiconductor switch?
An ideal semiconductor switch aims to balance performance with minimal electromagnetic interference (EMI). The challenges include managing fast edge rates that can cause unwanted oscillations and voltage spikes due to circuit parasitics. These issues can be addressed with good design practices and the use of snubbers.
How does the FET-Jet Calculator V2 assist in power design?
The FET-Jet Calculator V2 helps designers select SiC FETs and Schottky diodes by removing guesswork. It allows users to visualize device performance, facilitating quick and accurate first-pass designs.
What factors should be considered when selecting semiconductors for EV power converters?
Selection factors include the number and rating of parallel devices, switching frequency, operating mode, efficiency target, acceptable junction temperature rise, balancing conduction and switching loss, and cost.
What is the SEED methodology in ESD RF front-end design?
The SEED methodology involves using modeling and simulation to optimize an ESD RF front-end design, ensuring the mobile device’s ESD protection will pass system-level IEC stress testing before PCB layout.
How do advancements in semiconductor materials like GaN and SiC impact electronics?
GaN and SiC materials are revolutionizing power electronics and RF applications by offering improved performance, higher efficiency, and the ability to handle higher power densities and frequencies compared to traditional silicon-based semiconductors.
What is the importance of minimizing EMI and switching loss in SiC FETs?
Minimizing EMI and switching loss in SiC FETs is crucial for achieving efficient power conversion, enhancing electromagnetic compatibility (EMC), and unleashing the full potential of SiC devices without adding unnecessary complexity to designs.